The Journal of Advances in Parasitology
Review Article
Understanding of the Molecular Genetics of Benzimidazole Resistance in Haemonchus
Qasim Ali1*, Imran Rashid2, Kamran Ashraf2, Zubair Shabbir3, Umer Chaudhry4
1Department of Parasitology, Gomal University Dera Ismail Khan, Khyber Pakhtoon Khah, Pakistan; 2Department of Parasitology, University of Veterinary and Animal Sciences Lahore, Punjab, Pakistan; 3Quality Operations Laboratory, University of Veterinary and Animal Sciences, Lahore, Pakistan; 4University of Edinburgh, Royal (Dick) School of Veterinary Studies and Roslin Institute,Easter Bush Veterinary Centre, Midlothian, Scotland, EH25 9RG, UK.
Abstract | Parasitic infestations are continuously posing enormous threats to food security and poverty alleviation. While different drugs are proposed to minimize the impact of parasite, these come with established genetic resistance. Understanding markers of genetic resistance will streamline the process of use of alternative medication as well as assessing the affect of currently applied anthelmintic. This review briefly outlines the roles of parasites in diseases and proposes the use of different genetic markers to mitigate the emerging resistance in parasite.
Keywords | Haemonchus markers, Anthilmintic resistance, Resistance pattern
Editor | Muhammad Imran Rashid, Department of Parasitology, University of Veterinary and Animal Sciences, Lahore, Pakistan.
Received | June 26, 2019; Accepted | July 05, 2019; Published | April 25, 2019
*Correspondence | Qasim Ali, Department of Parasitology, Gomal University Dera Ismail Khan, Khyber Pakhtoon Khah, Pakistan; Email: qasim8485@gmail.com
Citation | Ali Q, Rashid I, Ashraf K, Shabbir Z, Chaudhry U (2019). Understanding of the molecular genetics of benzimidazole resistance in haemonchus. J. Adv. Parasitol. 6(2): 24-34.
DOI | http://dx.doi.org/10.17582/journal.jap/2019/6.2.24.34
Copyright © 2019 Ali et al. This is an open access article distributed under the Creative Commons Attribution License, which permits unrestricted use, distribution, and reproduction in any medium, provided the original work is properly cited.
In previous few decades, the revolution of the modern molecular techniques happened. These modern advances have genuine ramifications of different zones of Parasitology, examples are the expansion of the modern techniques for the prevention of parasitism through a higher learning of parasitic reproduction and improvements (Gasser, 2006; Hodgkinson, 2006). Likewise, the correct distinguishing proof of parasites (without the expense of sexual and developmental stage) and the inherited characterization have fundamental ramifications for taxonomy (scientific order and phylogenetic relationship), people hereditary qualities, science and the investigation of sickness transmission, and are furthermore vital to examination, medication and the prevention of the disease that they cause (Conraths and Schares, 2006; Gasser, 2006).Generally, the clinical symptoms of infection with bursate (order Strongylida) roundworms have been established on the identification of eggs and larvae from the feces of the animal. The presences of the eggs of various types of strongylids are the genuine diagnostic issues, for example, the eggs of hookworms, trichostrongyloids and knob worms (Setaria digitata) have similar basic morphology (except for those of the class Nematodirus). To overcome this problem, the culture of the feces is used to transform the eggs to infective larvae L3, which help the identification of parasite at the genus level. Nevertheless, coprological studies using this methodology, is troublesome, monotonous and requires individual abilities at perceiving these developmental stages (Gasser, 2006; Jacobs and Schnieder, 2006). Nevertheless, these criteria are much of the time lacking for particular identification. Diverse nucleic acid procedures give different techniques to beat the imperatives of standard systems, mainly for those who depend upon the DNA amplification (Monis et al., 2009). Furthermore, the DNA sequence based amplification, ligase reaction, displacement amplification, replicas-mediated amplification and linear-L amplification (Conraths and Schares, 2006). The polymerase chain reaction (PCR)based techniques are very helpful, accurate
Table 1: Antihelminthdrugs resistance status of different countries.
Countries | Benzimidazoles (route) | Imidazothiazoles (route) | Macrocyclic lactones (route) | Nematode species/genera |
Argentina | Oral,Inj | Oral ,Inj | Inj | Hspp,Cspp,Ospp, |
Australia | Oral | --- | oral | Hspp,Cspp,TSSP, |
Brazil | Inj | Inj | Inj | Hspp,Cspp,Ospp, |
Belgium | - | Inj | Inj | Cspp,Ospp, |
United Kingdom | - | - | Inj | Cspp, |
Germany, | - | - | Inj | Cspp,Ospp, |
Sweden | - | - | Inj | Cspp,Ospp, |
Belgium, | - | - | Inj | Cspp,Ospp, |
India | Morantel tartrate (oral) | Hp | ||
New Zealand | Oral | - | Oral ,Inj | Hspp,Cspp,Ospp,Tspp |
USA | Oral ,Inj | - | Inj |
Hspp,Cspp,Ospp, |
(Hspp= Haenonchus species, Cspp= Cooperia species, Ospp= Ostertagia species, Tspp= Tricostronglidaespecies, Hp= Haemonchus placei)
and easy accusable for the analysis purpose (Gasser, 2006).
Antiparastic Drugs and Development of Their Resistance in Livestock
GI nematode infections are causing the significant diseases in the animals of Pakistan and around the world. A viable technique is required for the successful control of nematodes by reducing their disease rate and transmission to other host keeping in mind that the end goal is to shield dairy cattle from production disasters. Procedures for the control of roundworms by utilizing antihelmintic medications must to be formulated in light of the quantization and identification of species (Mochizuki et al., 2006). There are five broad-spectrum and one narrow spectrum anthelmintic classes but mostly three classes of anthelmintic medications; Benzimidazoles (BZ), Imidazothiazoles (IT) and Macrocyclic lactones (ML) are utilized for the control of nematode parasites around the world. Antiparasitic drugs resistance has been characterized as a lessening in the adequacy of a medication against a populace of parasites, which are typically powerless to this medication by a particular dosage or focus. Multiple resistance develop when the entities are resistance to at least 2 diverse anthelmintic classes either because of selection by each class autonomously or because of cross-resistance (Prichard et al., 1980). Resistance inside a medication class has a tendency to be normal to all individuals from the class, in spite of the fact that distinctions in strength imply that a few medications hold action nevertheless when obvious resistance has been recorded to others in their group.
Resistance from each of the three classes, benzimidazoles, levamisole and the avermectins, have now been accounted for in various parts of the world, and nematode parasite populaces have been found with resistance from every one of the three main classes of drugs (McKellar and Jackson, 2004). Published cases of anthelmintic resistance in different country of the world in gastrointestinal nematodes of cattle illustrated below in Table 1 and Table 2 (Sutherland and Leathwick, 2011).
Nematodes infections are controlled in ruminants which mainly depends upon the use of broad spectrum anthelmintic prophylactically (McKellar and Jackson, 2004). Anthelmintic resistance to the all main classes has major negative impact on animal prosperity and production proficiency making control gradually more difficult and in some cases unmanageable (Gilleard, 2006; Kaplan, 2004). Multidrug resistance now impedes the sustainability of small ruminant industry. On the other side, despite a worldwide concern in the progress of antihelmintic resistance in small ruminants, only slight attention is given to the progress of drug resistance in large ruminant parasites (Coles, 2002). There are sluggish progresses in development of resistance in large ruminants as compared to the small ruminants, where it is very rapid. But now reports from the different part of world show that anthelmintic resistance in cattle parasites is emerging increasingly (Gasbarre et al., 2009a; Gasbarre et al., 2009b; Sutherland and Leathwick, 2011) and indicate a significant challenge to the cattle industry. However the knowledge of genetics and mechanism of drug resistance development is now succeeding but till there are much more facts that remain poorly understood (Beech et al., 2011).
Phenotypic and Genotypic Assays used for the Anthelminthic Resistance Diagnosis
Dynamic issue of anthelmintic resistance has provoked the expansion understanding of the requirement for dependable and consistent identification strategies (Hunt et al., 2008). Phenotypically the most valuable methods including, fecal egg reduction test, egg hatching assay and the coprological test, number of disadvantages regarding price, fittingness, and comprehension of perception were observ-
Table 2: Anthelmintic resistant percentage reported in world (Ramos et al., 2016).
Drugs | New Zealand | Brazil | Argentina | Belgium, Germany and Sweden | Belgium and Germany |
% Benzimidazoles (BZ) Resistance | 76% | 25% | 32% | Not detected | Not detected |
% Imidazothiazoles (IT) Resistance | 6% | 8% | Not detected | Not detected | Not detected |
% Macrocyclic lactones (ML)Resistance | 92% | 92% | 60% | 74% | 39% |
% Multidrug Resistance | 74% | 8-12% | 28% | Not detected | Not detected |
ed during phenotypic identification. Furthermore, these strategies are similarly tedious and labor required. To overcome these issues, molecular techniques are used for the finding of antihelmintic medication resistance. These advance techniques includes SNP-PCR, qPCR, direct sequencing, pyrosequencing genotyping and next generation sequencing(deep amplicon sequencing etc.) (de Lourdes Mottier and Prichard, 2008). Isotype-1 gene of the beta-tubulin, NA gated cation channels and GABA gene were genetically changed which leads to the development of resistance in roundworms parasite (Beech et al., 2011; de Lourdes Mottier and Prichard, 2008), so by employing the above genetic marker, we can identify the anthelmintic resistance.
Biology of Haemonchus
A standout amongst the most important group of GI parasitic nematodes of domestic livestock belongs to the genus Haemonchus (superfamily Trichostrongylidea).Haemonchus has four species (i-e) Haemonchusplacei, Haemonchus contortus, Haemonchussimilis and Haemonchus longistipeswhich were reported in different areas of the world indifferent hosts. The roundworm;Haemonchus is a blood sucking parasite that lives in the abomasa (or stomach) of different artiodactyl hosts (Hoberg et al., 2004) and they are among the most financially critical helminth parasites of bovine, sheep and goats around the world (Urquhart et al., 1996). The development birthplace of genus Haemonchusis sub-Saharan Africa where a mixture of animal types in native artiodactyls hosts are available. Three of these H. placei, H. contortusand H. similis are more universally transported by the anthropogenic movement of local domesticated animals.H. placei is dominating parasite of bovine and of major financial significance in tropical and sub-tropical areas (Urquhart et al., 1996). It presents sporadically in moderate areas, for example, Europe (western), Asia and recently it has also been diagnosed in Sweden and Canada proposing temperature range extension. Resistance from both BZ and macrocyclic lactones has been observed in H. placei, however generally little data is available on its prevalence (Chaudhry et al., 2014). The sympatric nematode H. contortusis a profoundly pathogenic nematode species that infect extensive of ruminant species however is most prevalent in sheep and goats. In spite of the fact that H.contortus is initially a tropical nematode, however recently it is reported in numerous mild and even sub-ice regions with continuous range expansion potentially connected with a dangerous atmospheric global warming (Waller et al., 2004). Haemonchus contortus is the most drug resistant parasite worldwide including multi-drug resistance, making its control progressively troublesome (Gilleard, 2006; Kaplan, 2004).
H.contortus and H.placei association
A number of early discussions in regards to the connection among H.placei and H.contortus. During late 70s, Gibbons (1979) thought about H.placei and H.contortus which belong to single species not two. But the morphological and molecular analysis supported these isolate into separate species. Many fixed differences of the morphology like pattern of cuticle ridges and also the spicule pattern were different in both species (Jacquiet et al., 1997; Lichtenfels et al., 1986). Other differences include image of the stained metaphase, spreads of karyotype through DAPI methods; all chromosomes (autosomes, sexsomes) are of equal size in case of H.contortus, while the sexosomes are larger than autosomes in case of H.placei (Amarante et al., 1997; Le Jambre, 1979). Moreover, there are fixed sequencing contrasts in the rDNA (ITS-2), rDNA (NTS) and mtDNA (nd4) gene (Blouin et al., 1997; Stevenson et al., 1996; Zarlenga et al., 1994).
H.contortusresistance Model Parasite
Antihelminth resistance is an important issue for the animal parasites control and a possible risk to the maintainability of group wise treatment programs being utilized for the control human parasitic infections in the emerging countries. Antihelmintic resistance is basically a quantitative complex quality by which different transformations take place to change the resistance phenotype. Essentially, mixes of bioinformatics and genetic approaches are required to perceive the principal changes and measure the influence of the resistance makeup. Thus, a model parasite is required as a key to the other parasite species for genetic and genomic approaches. Haemonchus contortus is a small ruminant nematode parasite; who has demonstrated an amazing desire to create resistance from any of the medications utilized as a part of its control. Incompletely along these lines, and somewhat as a result of its test manageability, inquire about on this nematode has donated more knowledge of resistance against the antihelmintic drugs than the any other parasite. H.contortus deals range of preferences as a trial framework comprising the capacity for attempt hereditary crosses; a precondition for hereditary mapping. This analysis will talk about the present advance on creating H. contortus used as a model more easily understandable in terms of resistance study than the other parasite (Gilleard, 2013).
Genetic Structure of H. contortus
Roundworm mtDNA experiences a great rate of genetic changes and the rate of change in Haemonchus contortus is ten times high as compared to the vertebrates. The DNA of H.contortus has a great degree of diversity. DNA of the H.contortus contains different genetic subunits like microtubules, different glycol proteins, a pair of glutamate cl-channel subunits, acetylcholine receptors, PEP carboxykinase, galectins, Tc1 transposable component, transposon and microsatellites repeats. All above hereditary investigations showed great genetic variety (Anderson et al., 1998; Kwa et al., 1993). Genetic variety may be intra-population or inter-populations (topographically isolated or anthelmintic selection). Experiment in four types of trichostrongylid roundworms showed that 96 to 99% variation of nucleotides are found in intra-populations (Hoekstra et al., 1997). H. contortus indicates awesome hereditary mutation both intra-populations and inter-population. Polymorphism relies upon the rate of transformation, the reasonable population size and the rates of movement. H. contortus is a great fruitful nematode present in large numbers of ruminants from the humid to slight cool atmospheres. Its host population estimate is immense due to the domestication of ruminants, and selling the animals’ is major resource of relocation of the parasite. Up to thousands of H. contortus worms can be present in single animal. This worms a productive reproducer that is a single female produced up to 10000 eggs. The size of the Population on field is typically substantially higher as compared to the inside of animal. The successful population size is enormous in H. contortus. The estimate of the population, its high multiplication level and the huge scope of its condition are helpful for extraordinary hereditary mutation. Benzimidazoles (BZs) binds to beta-tubulin, resulting in the disturbance of microtubules synthesis phenomenon. Genetic variation or mutations on the gene of β-tubulins were observed which modified the phenotype of H. contortus from the susceptible to resistance (Grant, 1994; Prichard, 2001).
Benzimidazole Mode of Action in Haemonchus
During the 60s, the first drug of BZ group, thiabendazole, was discovered and along these lines various anthelmintic of the BZ class have been presented and stay in regular use for the control of helminths programs in domesticated and friendly animals (McCracken and Lipkowitz, 1990). The mode of activity of BZ was at first resolved to incorporate the weakening of microtubule function. Microtubules are a crucial portion of the cytoskeleton filling a decent gene of the interest comprising improvement in the chromosomes in the middle of division of the cell, giving a supporting structure to the cell and an advancement of intracellular particles framework and exocytosis. The 450 amino acids are used in the formation of five microtubules proteins known as one alpha- tubulin and 4 beta-tubulin. The microtubules are formed by a dynamic process in which monomers of the tubulin are joined positive side and other negative side depolemerization occur. Beginning examinations on the method of activity of the BZ in nematodes were in Ascaris where BZ disrupts the microtubules of the intestinal cells of microtubule work (Perry and Randolph, 1999; Van den Bossche et al., 1982). In this manner, microtubules of the intestinal cells in parasitic nematodes Trichostrongylus vanished after treatment of BZ. Be that as it may, when a resistant population treated similarly the microtubules were as yet present (Sangster et al., 1985). Genetic analysis of the above phenomenon, it was assumed that BZ binds with microtubules to apply its impact on the monomers tubulin protein formation and interrupt the development of β-tubulin (Friedman and Platzer, 1978). Microtubulin molecule have 3 main drug tying positions, the vinblastine restricting site, the taxol restricting site and the colchicine restricting site (Gill and Lacey, 1992). The benzimidazole drugs bind at colchicine restricting site and interrupt the polymerization of β-tubulin monomer in roundworms parasite (Dawson et al., 1984; Friedman and Platzer, 1978; Sangster et al., 1985) by making a complex of benzimidazole and tubulin,inhibiting the fusion of tubulin monomers at positive shaft which ultimately hinder the formation and preservation of β-tubulin. Ultimately the starvation of nematodes occur by disruption of the intestinal cell (Abongwa et al., 2017) (Figure 1). Above research work assisted this theory of stopping of the polymerization of beta tubulin in vivo as well as in vitro (Lubega et al., 1993; Oxberry et al., 2001a; Oxberry et al., 2001b).Diagrammatically, model was constructed about the mode of action of benzimdazole drugs in H. contortus (Prichard, 2001; Robinson et al., 2004).
Mechanism of Action of Benzimidazole Resistance in Haemonchus
In the beginning of 80s, Driscoll discovered in the free living roundworm Caenorhabditis elegans through mutagenesis to perceive inherited loci that could develop BZ resistance. Overall, twenty eight mutations were recognized in C. elegans that involve in BZ resistance development and these present on a single locus and ben-1. Genetic cloning developed for the encoding of the β-tubulin ben-1 (Driscoll et al., 1989). In the beginning of 90s, first time in H. contortus β-tubulin locus was observed with the help
of southern blots and representing a diminishing in the amount of hybridizing areas in resistance differentiated risky populations (Geary et al., 1992; Kwa et al., 1993; Lubega et al., 1994; Roos et al., 1990). In a comparative
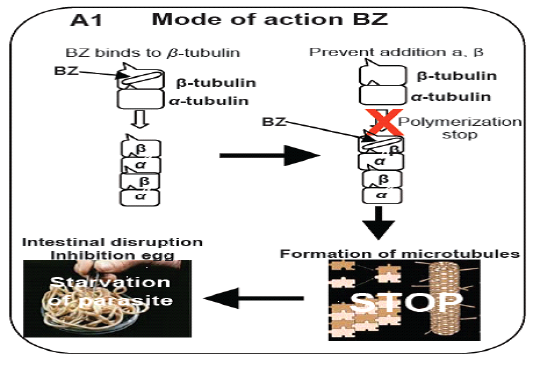
Figure 1: Model illustrates the mechanism of action of benzimidazole (BZ) drug (Prichard, 2001; Robinson et al., 2004).
study, cloned pieces of isotyp-1 β–tubulin gene were used to test the polymorphism at said locus by using the southern blot technique and recognized the fragments of different length in H. contortus worm’s populations. On the bases of RFLP analysis of the resistance populations showed only 1 to 2 fragments and 2-6 fragments which were recognized for susceptible gene in H. contortus population based on restriction enzymes used for the study. This decrease in polymorphism proved that the isotype-1 locus of the beta-tubulin was responsible for production of resistance in parasitic populations (Roos et al., 1990). Likewise, when purified polypeptide from β-tubulin of susceptible populations of H. contortus showed strong binding to BZ in vitro while it displayed modified phenotypes for resistant populationsm (Lubega and Prichard, 1991). Further analysis of the sequence of the isotype-1 locus of the β-tubulin gene reveals that it is due to only the substitution of the single amino acid at position F200Y (TTC to TAC) was the principle polymorphism that decide the nature of action against the benzimdazole drugs in the populations of H. contortus (Kwa et al., 1993). Another study was published in which the deletion of the fragment from beta-tubulin gene of H. contortus was responsible for the development of resistance against benzimidazole and the amount of this type of resistance is extraordinary in nature (Beech et al., 2011). This is the only main case of such type of resistance in which isotype2 β-tubulin deletion was observed has been characterized along these lines its general significance is yet to be resolved. Above describe work gives solid proof that the phenylalanine to tyrosine substitution F200Y (TTC to TAC) is a vital determinant of BZ resistance (Figure 2). In any case, affiliation investigations of this compose can’t absolutely approve that a specific polymorphism is fit for giving a resistant appearance; it is believable that the polymorphism in gene could be practically accountable for resistance. To set up this connection among a SNP and the phenotypic resistant is a main task on account of nematodes parasite since there is an absence of opposite genetics to contemplate gene capacity in these organisms. Though, on account of BZ resistance, this obstacle was overwhelmed by a few investigations that limit the variation in the beta-tubulin gene to adjust BZ sensitivity was tried to utilizing the C. elegans for expression system as a hetero-locus gene (Kwa et al., 1995). The alleles of the isotype-1 locus of the β-tubulin that have the residues of the phenylalanine P200F (TTC) in H. contortus showed drug susceptibility while tyrosine deposit P200Y (TAC) alleles did not showed any drug susceptibility. Moreover, the practical importance of F200Y (TTC to TAC) substitution was specifically shown by test used for mutagenesis. Replacing the residue of phenylalanine with the residue of tyrosine at position P200Y (TAC) which evacuated the capacity of the alleles of β-tubulin collected from susceptible strain of the H. contortus return to ben-1ofC. Elegans mutant to resistant phenotype (Kwa et al., 1995). Starting now and into the foreseeable future, because of P200 change in isotype-1β-tubulin locus of H. contortus, believable relationship studies was run down by significantly examinations. Recently different changes have been observed in isotyp-1 β-tubulin genes which incorporate in the development of BZ resistance. The phenylalanine at position P167F (TTC) of β-tubulin isotype-1 was first reported in H. contortus which also have high affinity for the bind of benzimidazole with microtubules (Prichard, 2001; Robinson et al., 2004) & tyrosine residue P167Y (TAC) polymorphism in this position was thus, connected with BZ resistance (Prichard, 2001). This polymorphism incorporates a substitution at F167Y (TTC to TAC) in resistance parasite as like the position P200. The changes at both P167Y (TAC) and P200Y (TAC) have not been found in the equivalent allele sequence of H. contortus and appear to be fundamentally unrelated P167Y (TAC) was found just in worms that had the wild-type P200Y (TAC) (Redman et al., 2015; Sangster et al., 1985). Some later confirmation recommends that a glutamate to alanine substitution E198A (GAA to GCA) in isotype-1 locus of β-tubulin can conceivably add to BZ resistance. Ghisi (2007) and Rufener (2009) have recognized alanine substitution at codon P198A (GCA) in BZ resistance H. contorts populations. Likewise with P167Y (TAC), the P198A (GCA) polymorphism was discovered just in allelic sequence that was genetically wild-type at site P200Y (TAC) and P167Y (TAC) (Ghisi et al., 2007; Van den Brom et al., 2015).
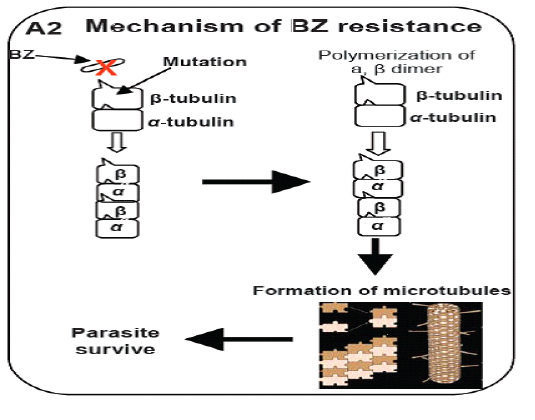
Figure 2: Model illustrates the mechanism of resistance of benzimidazole (BZ) drug (Prichard, 2001; Robinson et al., 2004).
Mechanism of BZ Resistance on Molecular Basis.
Above describe work for the component of benzimidazole resistance has been additionally inspected by hereditary means and solid confirmation exists that 3 unique SNPs [P200Y (TAC), P167Y (TAC) and P198A(GCA)] in the isotype-1β-tubulin gene was responsible for the resistance against benzimidazole (Figure 3) (Brasil et al., 2012; Chaudhry et al., 2016; Chaudhry et al., 2015b; Ghisi et al., 2007; Hoglund et al., 2009; Kotze et al., 2012; Kwa et al., 1994; Redman et al., 2015; Rufener et al., 2009; Silvestre and Humbert, 2002). Number of studies has demonstrated the P200Y (TAC) SNP happens in field populations of parasitic nematodes recommending far reaching significance around the world (Barrere et al., 2012; Barrere et al., 2013a; Barrere et al., 2013b; Brasil et al., 2012; Chaudhry et al., 2016; Chaudhry et al., 2015b; Hoglund et al., 2009; Redman et al., 2015; Silvestre and Humbert, 2002). The P167Y (TAC) SNP has been reported in various countries of the world, but it is less common than the P200Y (TAC) change (Brasil et al., 2012; Redman et al., 2015; Silvestre and Humbert, 2002). A SNP at codon P198A (GCA) has also been identified in populations oriented from field of parasitic nematode H.contortus (Chaudhry et al., 2015b).
Brief Overview Of Origin And Spread Of Benzimidazole Resistance
Although, good improvement has been made in explaining mechanisms of BZ resistance at molecular basis; but still there are many more debates. In particular there have only been a few published investigation about the origins and spread of resistant alleles against the benzimidazole drugs in the populations of parasites (Chaudhry et al., 2016; Chaudhry et al., 2015b; Redman et al., 2015; Silvestre and Humbert, 2002; Skuce et al., 2010). This is a complex topic but we suggest four broad models to determine how the drug resistance emerges and spreads in parasite populations (Fig. 2.6). These are obvious simplifications but in each case it allows different predictions to be made about the nature of the sequence polymorphism and the phylogenetic relationships between resistant alleles present in different parasite populations.
Model 1.
Resistance could arise as a “new” or recent mutation that originate from the single point and then spread in whole population or area as a result of animal movement. In model 1, a single resistant haplotype would sweep through the populations leaving a genetic footprint of reduced polymorphism about the hereditary locus due to selection that is termed as “hard selective sweep” (Figure 4 B1) (Pritchard et al., 2010). Model 1 is observed in different mosquitoes (Ramos et al., 2016) and Drosophila (Andreev et al., 1999) and BZ muted gene in H. contortus (Chaudhry et al., 2015b). In these cases the similar resistant haplotype is present in different resistant populations.
Model 2.
New mutations showing resistance might independently arise in different parasite populations with little or no migration between populations. In this case, there would be reduced polymorphism around the genetic locus under selection but different resistant haplotypes would be present in different populations (Figure 4 B2). This type of origin and spread of the resistance termed as “soft selective sweep”. Soft selective sweep was observed in BZ resistance alleles in several farms of goat in France which was dueto the movement of animals (Silvestre and Humbert, 2002).
Model 3.
New mutations showing resistance could independently arise in several (or even numerous) different locations and then convert stable by selection and transfer between parasite populations. The genetic footprint left by this has been named a “soft selective sweep” and is categorized not necessarily with a decrease in polymorphism about the locus but a region of linkage disequilibrium can still be potentially detected (Fig. 4 B3) (Pennings and Hermisson, 2006). This type of model was observed mutant genes for anthelmintics in T. circumcincta and H. contortusin the UK and Indian population (Chaudhry et al., 2016; Chaudhry et al., 2015b; Redman et al., 2015; Skuce et al., 2010) and observed in a number of insecticide resistance studies in different arthropods (Andreev et al., 1999).
Model 4.
Mutation could be conferred by an “ancient origin” as opposed to recent mutation(s). In this case, the polymorphism would have pre-existed in the ancestral parasite populations long before the onset of anthelmintic use. The polymorphism might be anticipated to be already present in most (if not all) parasite populations at some frequency even prior to drug selection (although the details of this would depend on the molecular bases of the worm population). Since the polymorphism is ancient, it would be anticipated to be present on multiple haplotype backgrounds due to effect of genetic recombination over the generations (Figure 4 B4). There would be potentially little genetic footprint of selection in this case. Instead, the genetic variation around the selected polymorphism would reflect the population genetic structure of the parasite population. This has been identified in parasitic nematodes by a few authors (Chaudhry et al., 2016; Roos et al., 1990; Silvestre and Humbert, 2002).
Figure 4: (B1, B2, B3, B4) is the schematic representation of four different models about the origin of resistance mutation in round worm populations. The blue circles represent different parasite populations at different geographical locations (e.g. different farms). The letter “R” represents a resistance mutation that is present in that parasite population and the colour of the letter represents the haplotype background upon which that mutation is present. Susceptible haplotypes are not represented for simplicity. The grey, double headed arrows represents gene flow among different parasite populations. (B1) In this model, a recent mutation arises either shortly before or during the period of anthelmintic selection in one geographical location and then spreads by migration between parasite populations. Following the drug selection, the frequency of the mutation would have increased and a single identical haplotype would be present in all populations. This extreme decrease in sequence polymorphism about the locus which is under selection is termed as “Hard selective sweep”. (B2) In B2 model, there is no gene flow between parasite populations. Mutations arise either during or shortly before the period of anthelmintic usage. In this case, there would be reduced polymorphism around the genetic locus under selection in each resistant parasite population but different resistance haplotypes would be present in the different populations. (Occasionally the same resistance haplotype might be found at different locations due to the chance of a genetic
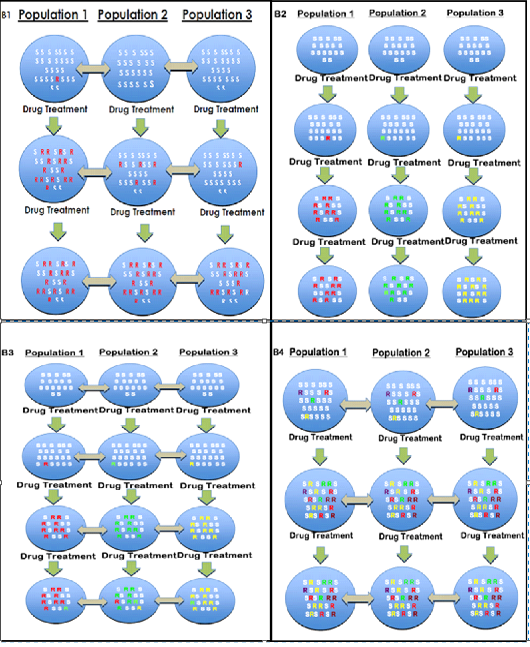
Figure 4 (B1, B2, B3, B4): Patterns of origin and spread of drug resistance (Chaudhry et al., 2016).
change is independently rising on the same susceptible codon that was shared between populations). (B3) This model is similar to that shown in B2 but in this case there is gene flow between parasite populations. Mutations arise at different geographical locations either during or shortly before the period of anthelmintic usage and then spread between parasite populations by migration. In this model, there will potentially be several (possibly numerous) different resistant haplotypes present with some shared and some different among different parasite populations. There is likely to be decreased polymorphism about the locus which is under selection (because susceptible haplotypes will be eliminated by drug treatment) but it is less extreme than for the model illustrated in panel B1. That’s termed as “soft selective sweep”. (B4) In this model an ancient pre-existing polymorphism is present in the parasite population and confers anthelmintic resistance. Since this mutation is present for a long time, possibly millions of years, it will be present in parasite populations on many different haplotype backgrounds due to the effects of recombination through the ages. Furthermore, for a parasite with little geographical sub-structuring the same haplotypes are likely present in the different parasite population at this locus. The frequency of resistance mutation increases after the drug selection. Hence, in this model, one might anticipate that the polymorphism is much less reduced round the locus under selection compared to the models shown in B1 to B3 and for numerous identical haplotypes to be present in the geographically separate parasite populations (although the details of this depends on the population structure of the parasite population).
References