Advances in Animal and Veterinary Sciences
Research Article
Potential Effects of Cranberry Extract Against Lead Acetate-Induced Hepato-Renal Toxicity in Rats
Hussein Ibrahim El-belbasy1, Mohammed Abdalla Hussein2, Mohamed El-mitwaly Alghitany3*
1Biochemistry Department, Faculty of Veterinary Medicine, Zagazig University-44519, Zagazig, Egypt; 2Biochemistry Department, Faculty of Applied Medical Sciences, October 6th University-12585, Giza, Egypt; 3Medical Laboratory Department, Faculty of Applied Medical Sciences, October 6th University-12585, Giza, Egypt.
Abstract | Lead (Pb) has been identified as a hazardous heavy metal and a pollutant in the environment, especially due to its human activity. It poisons several physiological systems, such as the hepatic, renal, reproductive, as well as nervous systems, because of an elevation in oxidative damage caused by the formation of reactive oxygen species (ROS). Cranberry is a powerful antioxidant in addition to being a component of an anti-inflammatory disease treatments. The goal of this study was to see if cranberry extract could protect rats from toxicity caused by lead acetate. Addition of cranberry extract at a dose of 75 and 150 mg/kg to rats allowed to treat with lead acetate at a dose of 50 mg/kg to 6 weeks significantly protected the rats from the lead acetate-induced increase in both serum alanine aminotransferase (ALT), aspartate aminotransferase (AST), lactate dehydrogenases (LDH), total and direct bilirubin, alkaline phosphatase (ALP), creatinine, urea, total cholesterol (TC), triglycerides (TG), LDL-C and VLDL-C in addition against an elevation of serum glucose, tumor necrosis factor-alpha (TNF-α) and malondialdehyde (MDA). Treatment with cranberry extract at a dose of 75 and 150 mg/kg also led to a valuable rise in serum total soluble protein, albumin, globulin, HDL-C, triiodothyronine (T3), total thyroxine (T4) as well as hepatic and renal tissue of reduced glutathione (GSH), superoxide dismutase (SOD), catalase activity (CAT) and total antioxidant capacity (TAC) as compared to lead acetate-treated rats. Cranberry has hepato-renal protective impacts in restoring liver and kidney function, according to histopathological evaluation of hepatic and renal tissues. These findings have shown, in conclusion, that cranberry extract has such a strong protective effect in rats suffering from hepato-renal toxicity caused by lead acetate.
Keywords | Cranberry extract, Lead acetate, Hepato-renal toxicity, Oxidative stress, Oxidative stress biomarkers
Received | June 02, 2021; Accepted | July 10, 2021; Published | August 25, 2021
*Correspondence | Mohamed El-mitwaly Alghitany, Medical Laboratory Department, Faculty of Applied Medical Sciences, October 6th University - 12585, Giza, Egypt; Email: [email protected]
Citation | El-belbasy HI, Hussein MA, Alghitany ME-M (2021). Potential effects of cranberry extract against lead acetate-induced hepato-renal toxicity in rats. Adv. Anim. Vet. Sci. 9(10): 1669-1683
DOI | http://dx.doi.org/10.17582/journal.aavs/2021/9.10.1669.1683
ISSN (Online) | 2307-8316; ISSN (Print) | 2309-3331
Copyright © 2021 El-belbasy et al. This is an open access article distributed under the Creative Commons Attribution License, which permits unrestricted use, distribution, and reproduction in any medium, provided the original work is properly cited.
INTRODUCTION
One of the most pressing issues confronting humanity today is environmental pollution (Ali and Khan, 2017). Numerous epidemiological studies have reported a strong relationship to human hepatic and renal damage and exposure to multiple environmental pollutants (Gao et al., 2015). Heavy metals enter the environment, pollute food supply and induce a variety of health issues because of their toxic effects. Prolonged exposure to such toxic metals puts living organisms in grave danger (Wieczorek-Da˛browska et al., 2013).
Lead is a great source of threat for public health as one of the most toxic environmental pollutants stable (Andjelkovic et al., 2019). According to Soliman et al. (2015), lead (Pb) has a high risk of causing hepatotoxicity as well as nephrotoxicity in most animal and human species. Because it is widely used in the production of materials like pigments, plastic batteries, fuel oil, cosmetics, tank connections, piping, poetry glassing, and dolls, it is regarded a significant toxic substance for the environment (ATSDR, 2007; WHO, 2010). The principal modes of exposure to lead in the ecosystem are through ingestion and inhalation of its particles, whereas in fewer cases it is through skin and prenatal exposure (Dapul and Laraque, 2014; ATSDR, 2017). Lead builds up in animal tissues like the liver, the kidney and the bones and these organs are often examined in wildlife toxicology experiments (Plaza et al., 2018).
Oxidative stress is defined as the difference between both the reactive oxygen species (ROS) formation and the antioxidants for free radicals (Costa et al., 2015; Fenga et al., 2016). The main toxic effects of lead are elevated ROS levels (Flora et al., 2012). This could occur by 2 distinct methods. The first route is the δ-aminolevulinic acid dehydratase (δ-ALAD) pro-oxidant effect. The Second pathway is linked to lead’s direct effect on cell membranes’ lipid composition (Ahamed and Siddiqui, 2007; Lopes et al., 2016). Latest studies reveal that reactive oxygen species (ROS) play an integral part in the nephrotoxicity caused by lead (Xu et al., 2008; Mervat et al., 2012). If lead damages the kidneys, it is referred to as “lead-related nephrotoxicity” by physicians. Throughout the renal tubules, lead is taken up by proximal tubules, in which many of the associated proteins associated with lead. Intercellular inclusions of proximal tubular cells are generated by these lead-binding proteins (Kwon et al., 2015).
Lead poisoning causes the normal anatomical organisation of hepatic lobules to be disrupted, as well as the disruption of the characteristic cord-like liver cells structure, hepatocytes that are hyperchromatic with transverse vacuoles, and congestion caused by sinuses (Bukola et al., 2015). According to Abdel Moneim (2016), Lead acetate caused primary hepatic cell death which was followed by the expansion of the sinuses in the blood and central veins congested, and acute inflammatory cells incursion, primarily throughout the core region, also the disorder sling liver cells with nuclei bloated nuclear, emptying liver cells, and change fatty (steatosis), that included fat accumulation within cells. Abdel Moneim (2016) and Shatha et al. (2016) found a notable rise in free radical production, liver transaminase activities (ALT and AST), as well as total bilirubin levels in male Wistar rats exposed to experimental lead-induced poisoning. Fluidity of the microsomal membrane in the liver, ROS formation, as well as hepatocytic histogram variation were all associated with increased serum ALT and AST action (Shatha et al., 2016).
Plants including such Flavonoids, rutines, glycosides, terpenoids, tannins and alkaloids are present in various kind of bioactive compounds, which act as antioxidants in stressful situations and have important protective properties such as antitumor, anti-inflammative, anti-mutagenic and immunomodular anti-hepatic toxicity (Blumberg et al., 2013). Cranberries are one of these plants, and they’ve long been prized for their medicinal properties. Native Americans used them to treat bladder and kidney problems. Cranberries contain a variety of bioactive compounds, including fructose, vitamin C, flavonoids, anthocyanidins, catechins, triterpenoids, and phenolic compounds, which are widely used for their health benefits and anti-cancer properties (Hisano et al., 2012).
Cranberry’s flavonoids scavenge free radicals, superoxide radicals, hydroxyl radicals, and lipid peroxidation, preventing mitochondrial destruction, degradation, and membrane integrity loss (Lapshina et al., 2015). Previous research has found that flavonoids in cranberry extract (CBE) have the ability to lower ALT and AST elevated concentrations and inhibit lipid membrane droplet buildup in rat liver models (Cheshchevik et al., 2012). Cranberry extract powder (CBE) is also used in food to boost the body’s antioxidant defenses.
The polyphenol compounds found in Vaccinium macrocarpon (American cranberry) possess tremendous and valuable properties against both infections, tumors, cancers and mutations. These polyphenols’ concentration in cranberries increases as the fruit ripens and the size of the fruit grows. Anthocyanins contain six aglycones. Phenolic acid, which includes hydroxybenzoic acid and hydroxycinnamic acid, is the second most prevalent compound in CBE. However, benzoic acid is found in significant quantities. Just three forms of hydroxybenzoic acid are found in smaller amounts: 2, 4-dihydroxybenzoic, p-hydroxybenzoic, and ohydroxybenzoic. Likewise, the hydroxycinnamic also includes different ingredients like pcoumar, ferulic acid, sinapic and caffeic. Terpenes are the third most plentiful bio-active compound but research on them has been limited compared to the polyphenolic composition. Flavonols can be found in cranberry fruit, but elderberry has a higher concentration (Hafez et al., 2014).
Cranberry extract’s protective function in lead acetate (LD)-induced hepato-nephrotoxicity has yet to be fully investigated. As a result, the objective of this work was to evaluate the protective and therapeutic potential effects of cranberry extract in rats with hepato-renal toxicity caused by lead poisoning.
MATERIALS AND METHODS
Chemicals and other reagents
Company of Sigma Chemical supplied the lead acetate (St. Louis, MO, USA). Prior to administration, the lead was diluted with distilled water. The other chemicals utilized in this investigation were all analytical quality, kept in a controlled environment, and obtained from well-known commercial sources.
Plant material
Virgin Extracts (TM), China, provided the cranberry water extract (CBE). Cranberry was supplied a period of six weeks to adult rats daily via oral gastric gavage tube at a dose of 75 mg/kg (1/150 LD50) and 150 mg/kg (1/75 LD50).
CBE analysis by gaseous chromatography/ mass spectrometer (GC–MS)
At the Mycology and Biotechnology Regional Center, Al-Azhar University, in Nasr city, Cairo, Egypt, the cranberry extract chemical composition was determined using a detectable GC1310-ISQ mass spectrometer (Thermo Scientific, Austin, TX, United states). A direct capillary column TG–5MS (30 m x 0.25 mm x 0.25 m film thickness) was used in the GC–MS scheme. At first, the temperature of column oven was maintained at 50°C, after which increased at a rate of 5°C/min to 230°C, held for two minutes, and then boosted at a rate of 30°C/min to 290°C, held for two minutes. Temperatures for injection and MS transmission lines were maintained at 250 and 260 degrees Celsius, respectively, and Helium was utilized as a carriage gas at a regular flow speed of 1 ml/min. The solvent time limit was three minutes and the Auto sampler AS1300 injected 1μL diluted samples automatically in the split mode together with GC. In full scan mode, EI mass spectrum was captured over the range of m/z 40–1000 at 70 eV ionization voltages. The temperature of the ionization chamber was established to 200°C. By comparing the stability times and mass spectrometry of the components to those of the WILEY 09 and NIST 11 mass spectral databases, the elements were detected.
Animal study
Ethical issues
The present study has already been authorized by the Zagazig University’s institutional Animal Care and Use Committee with the authorization number (ZU- IACUC/2/F/169/2019).
Animals
The experimental investigation of this study used a total number of 40 male adult albino rats weighing approximately 200 ± 20 gms, obtained from the Central Animal House, Faculty of Veterinary Medicine, Zagazig University. They were allowed to acclimatize for 2 weeks. They were kept at 22±2℃ and humidity of 60% for a 12-hours light-dark period in stainless steel cages in a controlled environmentally-conditioned area. During the adaptation phase, each animal was fed an ad libitum diet (Dyets Inc., Bethlehem, PA) and had free access to water.
Experimental design
After acclimatization, rats were divided into four groups (10 rats per group) and given an oral daily dose by gavage method of various treatments.
Group I (normal control group)
Each rat was given 1 ml of distilled water orally for 6 weeks (Abdel-Maksoud et al., 2015a).
Group II (positive control group)
Each rat was given lead acetate at a dose of 50 mg/kg orally for 6 weeks (Sri et al., 2017).
Group III
Each rat was given lead acetate at a dose of 50 mg/kg followed by cranberry extract suspended in distilled water at a dose of 75 mg/kg for 6 weeks (Abdel-Maksoud et al., 2015a).
Group IV
Each rat was given lead acetate at a dose of 50 mg/kg followed by cranberry extract suspended in distilled water at a dose of 150 mg/kg for 6 weeks (Abdel-Maksoud et al., 2015a).
Blood samples
After the experiments ended, each group of rats was fasted nightly, weighted, then euthanized via cervical dislocation. Blood was drawn in glass test tube (without EDTA) and left at the room temperature to coagulate for 20 min until centrifugation on 3000 rpm for ten min. The serum has been carefully obtained and deposited at -20°C until biochemical tests have been further used (in 2 weeks). The serum that was collected was used to estimate serum alanine aminotransferase (ALT) (Reitman and Frankel, 1957), aspartate aminotransferase (AST) (Reitman and Frankel, 1957), lactate dehydrogenases (LDH) (Buhl and Jackson, 1978), total and direct bilirubin (Mashige et al., 1981), alkaline phosphatase (ALP) (Tietz, 1983), total soluble protein (Yatzidis, 1977), albumin (Doumas and Biggs, 1972), globulin, creatinine (Henry, 1974), urea (Batton and Crouch, 1977), total cholesterol (TC) (Allain et al., 1974), triglycerides (TG) (Fossati and Prencipe, 1982), HDL-C (Shih et al., 2000), LDL-C (Friedewald et al., 1972), VLDL-C (Friedewald et al., 1972), glucose (Trinder, 1969), triiodothyronine (T3) (Tammas and Daniel, 2009), total thyroxine (T4) (Berbel et al., 2010), and tumor necrosis factor-alpha (TNF-α) (Beyaert and Fiers, 1998) levels.
Preparation of liver and renal samples
After collecting blood samples, the liver and kidneys were removed and rinsed in sodium chloride solution (0.9%) then subdivided into two sections.
Histological assessment
Specimens from the rats liver and kidney have been taken then preserved inside a 15% neutral-buffered formalin solution for 48 h, dried in progressively rising ethanol (from 70 to 100%), cleaned in xylene, then inserted in paraffin-wax. A microtome was used to cut down five µm thickness paraffin (Leica RM 2155, England). The last step, the slides had been obtained then normally dyed using hematoxylin and eosin (HE) stains (Suvarna et al., 2018). The images had been captured with a Leica® microscopy and an AmScope® digital microscope camera. Lesions Score system was created as follows: system (-= -No Alterations 0%, += Mild Alterations 10-35%, ++= Moderate Alterations 40-50%, +++= Severe and ++++= Intense Alterations up to 60%).
Analytical statistics
SPSS version 25 was used to analyze all of the data (Armonk, NY: IBM Corp). The data have been shown as a mean ± SE. For certain parameters, a one-way ANOVA was used to see if there were any differences between groups. The post-hock test was done using Duncan’s multiple range test. It was statistically significant if P < 0.05.
RESULTS AND DISCUSSION
CBE analysis by gaseous chromatography/mass spectrometer (GC–MS)
The Gas chromatography-mass results for cranberry extract oil samples, displayed in (Table 1), which revealed qualitative and quantitative variation in the chemical composition. In cranberry extract compounds were identified which accounted for 29.33% of stearic acid, 10.44% Palmitic acid, 3% Oleic acid, 7.59% Myristic acid, 6.94% furfural, 4.84% marphinan, 5.05% tridemorph, 3.81% oxirane, 1.68% docosane, 1.7% lycopene, 19.97% Methyl furfural, 2.56% D-glucital, 1.5% glycan, 2.5% Dimethyl propanol and 1.22% siloxane.
Table 1: Chemical composition of cranberry extract.
RT | Compound Name | % |
5.28 | Stearic acid | 29.33 |
6.41 | Palmitic acid | 10.44 |
6.74 | Furfural | 6.94 |
10.73 | Morphinan | 4.84 |
13.92 | Tridemorph | 5.05 |
15.75 | 0xirane | 3.81 |
15.94 | Docosane | 1.68 |
18.02 | Lycopene | 1.7 |
19.30 | Methyl furfural | 19.97 |
21.71 | D-glucital | 2.56 |
23.45 | Glycan | 1.5 |
27.17 | Myristic acid | 7.59 |
35.23 | Dimethyl propanol | 2.5 |
39.46 | Oleic acid | 3 |
57 | Siloxane | 1.22 |
Effects on serum biochemical assays
The results displayed in (Table 2) revealed a significantly difference in serum alanine aminotransferase (ALT), aspartate aminotransferase (AST), lactate dehydrogenase (LDH), alkaline phosphatase (ALP), total soluble protein, albumin and globulin levels (P< 0.001) and also in serum total and direct bilirubin levels (P< 0.0001) between the four groups treated. In comparison with normal control group, oral intake of lead acetate at a dose of 50 mg/kg led to a substantial rise in serum alanine aminotransferase (ALT), aspartate aminotransferase (AST), lactate dehydrogenase (LDH), total bilirubin, direct bilirubin and alkaline phosphatase (ALP) levels, and also a substantial reduction in the levels of total soluble protein, albumin and globulin in serum. In comparison to the group that received lead acetate, addition of 75 and 150 mg/kg cranberry extract led to a marked decrease in serum alanine aminotransferase (ALT), aspartate aminotransferase (AST), lactate dehydrogenase (LDH), total bilirubin, direct bilirubin, alkaline phosphatase (ALP) levels as well as a marked rise in total soluble protein, albumin and globulin levels in serum.
The findings in (Table 3) indicated a significantly difference in serum creatinine (P< 0.001) as well as serum urea (P< 0.0001) levels among the four treated groups. In comparison with normal control group, oral intake of lead acetate at a dose of 50 mg/kg led to a substantial rise in serum creatinine and urea levels. In comparison to the group that received lead acetate, addition of 75 and 150 mg/kg cranberry extract led to a marked decrease in serum creatinine and urea levels.
The data of (Table 4) demonstrated there had been a highly significant variation in serum total cholesterol (TC), triglycrides (TG), HDL-C, LDL-C as well as VLDL-C levels among the four treated groups (P< 0.001). In comparison with normal control group, oral intake of lead acetate at a dose of 50 mg/kg led to a substantial rise in serum total cholesterol (TC), triglycrides (TG), LDL-C and VLDL-C levels. Also, the level of HDL-C in serum has decreased significantly. In comparison to the group that received lead acetate, addition of 75 and 150 mg/kg cranberry extract led to a marked decrease in serum total cholesterol (TC), triglycrides (TG), LDL-C and VLDL-C. Also, the level of serum HDL-C has increased significantly.
Table 2: Effect of cranberry extract on serum alanine aminotransferase (ALT), aspartate aminotransferase (AST), lactate dehydrogenases (LDH), total and direct bilirubin, alkaline phosphatase (ALP), total soluble protein, albumin and globulin levels in normal and experimental groups of rats.
Groups parameters | Normal group | Positive control (lead acetate 50 mg/kg.b.w.) | Lead acetate (50 mg/kg.b.w.) + Cranberry extract (75 mg/kg) | Lead acetate (50 mg/kg.b.w.) + Cranberry extract (150 mg/kg) |
ALT (U/L) |
16 ± 0.70d |
63 ± 2.82a |
44.60 ± 1.86b |
27.40 ± 2.01c |
AST (U/L) |
21.40 ± 1.02d |
71.60 ± 4.11a |
60 ± 2.38b |
38 ± 1.87c |
LDH (U/L) |
1120 ±9.88d |
1530.6±31.05a |
1378.2±10.24b |
1241.8±18.30c |
Total bilirubin (mg/dl) |
2.61 ± 0.07d |
4.98 ± 0.16a |
4.01 ± 0.07b |
3.26 ± 0.14c |
Direct bilirubin (mg/dl) |
0.41 ± 0.02d |
2.02 ± 0.05a |
1.34 ± 0.06b |
0.87 ± 0.02c |
ALP (IU/L) |
68 ± 2.12d |
111.80 ± 2.70a |
95.40 ± 1.77b |
80 ± 1.81c |
T.proteins (g/dl) |
7.49 ± 0.20a |
4.18 ± 0.18d |
5.89 ± 0.06c |
6.78 ± 0.14b |
Albumin (g/dl) |
3.95 ± 0.076a |
1.99 ± 0.025d |
2.75 ± 0.036c |
3.15 ± 0.038b |
Globulins (g/dl) |
3.53 ± 0.13ab |
2.19 ± 0.18c |
3.14 ± 0.07b |
3.63 ± 0.17a |
abcd Means with different superscript within same row are statistically different at level P<0.05 according to Duncan’s multiple range test.
Table 3: Effect of cranberry extract on serum creatinine and urea levels in normal and experimental groups of rats.
Groups parameters | Normal group | Positive control (lead acetate 50 mg/kg.b.w.) | Lead acetate (50 mg/kg.b.w.) + Cranberry extract (75 mg/kg) | Lead acetate (50 mg/kg.b.w.) + Cranberry extract (150 mg/kg) |
Creatinine (mg/dl) |
0.77 ± 0.022d |
1.93 ± 0.007a |
1.31 ± 0.039b |
0.98 ± 0.011c |
Urea (mg/dl) |
23.24 ± 0.80d |
53.38 ± 0.99a |
43.04 ± 1.06b |
34.78 ± 0.83c |
abcd Means with different superscript within same row are statistically different at level P<0.05 according to Duncan’s multiple range test.
Table 4: Effect of cranberry extract on serum total cholesterol (TC), triglycrides (TG), HDL-C, LDL-C and VLDL-C levels in normal and experimental groups of rats.
Groups parameters | Normal group | Positive control (lead acetate 50 mg/kg.b.w.) | Lead acetate (50 mg/kg.b.w.) + Cranberry extract (75 mg/kg) | Lead acetate (50 mg/kg.b.w.) + Cranberry extract (150 mg/kg) |
TC (mg/dl) |
91.6000 ± 2.01494d |
141.80 ± 1.88149a |
120.80 ± 1.24097b |
103.00 ± 3.27109c |
TG (mg/dl) |
67.0 ± 2.93c |
101 ± 2.46a |
87.60 ± 2.15b |
80.8 ± 1.35b |
HDL-C (mg/dl) |
54.80 ± 1.77a |
27.40 ± 1.20d |
39.60 ± 1.86c |
49.60 ± 1.88b |
LDL-C (mg/dl) |
23.40 ± 1.76d |
94.20 ± 2.92a |
63.68 ± 2.71b |
37.24 ± 4.76c |
VLDL-C (mg/dl) |
13.44 ± 0.60c |
20.20 ± 0.49a |
17.52 ± 0.43b |
16.14 ± 0.26b |
abcd Means with different superscript within same row are statistically different at level P<0.05 according to Duncan’s multiple range test.
The results displayed in (Table 5) showed a significantly difference in serum glucose, triiodothyronine (T3), total thyroxine (T4) as well as tumor necrosis factor-alpha (TNF-α) levels among the four treated groups (P< 0.001). In comparison with normal control group, oral intake of lead acetate at a dose of 50 mg/kg led to a substantial rise in serum glucose and tumor necrosis factor-alpha (TNF-α) levels as well as a significant reduction in serum triiodothyronine (T3) and total thyroxine (T4) levels. In comparison to the group that received lead acetate, addition of 75 and 150 mg/kg cranberry extract led to a marked decrease in serum glucose and tumor necrosis factor-alpha (TNF-α) levels as well as a marked rise in serum triiodothyronine (T3) and total thyroxine (T4) levels.
Effect on antioxidant parameters in hepatic and renal tissue
The findings in (Table 6) indicated a significantly difference in reduced glutathione (GSH) level, superoxide dismutase (SOD), catalase activity (CAT), malondialdehyde (MDA) and total antioxidant capacity (TAC) in hepatic and renal tissue of the four assessment groups where (P< 0.001). In comparison with normal control group, oral intake of lead acetate at a dose of 50 mg/kg led to a marked reduction in reduced glutathione (GSH) level, superoxide dismutase (SOD), catalase activity (CAT) and total antioxidant capacity (TAC) of hepatic and renal tissues. Also, the level of malondialdehyde (MDA) has increased significantly in hepatic and renal tissues. In comparison to the group that received lead acetate, addition of 75 and 150 mg/kg cranberry extract led to a marked development in reduced glutathione (GSH) level, superoxide dismutase (SOD), catalase activity (CAT) as well as total antioxidant capacity (TAC) in hepatic and renal tissue. Also, the level of malondialdehyde (MDA) has decreased significantly in hepatic and renal tissues.
Table 5: Effect of cranberry extract on serum glucose, triiodothyronine (T3), total thyroxine (T4) and tumor necrosis factor-alpha (TNF-α) levels in normal and experimental groups of rats.
Groups parameters | Normal group | Positive control (lead acetate 50 mg/kg.b.w.) | Lead acetate (50 mg/kg.b.w.) + Cranberry extract (75 mg/kg) | Lead acetate (50 mg/kg.b.w.) + Cranberry extract (150 mg/kg) |
Glucose (mg/dl) |
86 ± 2.44d |
162 ± 2.77a |
132 ± 3.44b |
107.8 ± 2.81c |
T3 (ng/ml) |
0.438 ± 0.04a |
0.101 ± 0.01d |
0.178 ± 0.01c |
0.331 ± 0.01b |
T4 (nmol/L) |
11.52 ± 0.27a |
2.42 ± 0.13d |
5.34 ± 0.25c |
8.86 ± 0.10b |
TNF-α (pg/ml) |
2.13 ± 0.080d |
12.61 ± 0.43a |
8.02 ± 0.11b |
5.18 ± 0.06c |
abcd Means with different superscript within same row are statistically different at level P<0.05 according to Duncan’s multiple range test.
Table 6: Effect of cranberry extract on reduced glutathione (GSH), superoxide dismutase (SOD), catalase activity (CAT), malondialdehyde (MDA) and total antioxidant capacity (TAC) levels in hepatic and renal tissue of normal and experimental groups of rats.
Groups parameters | Normal group | Positive control (lead acetate 50 mg/kg.b.w.) | Lead acetate (50 mg/kg.b.w.) + Cranberry extract (75 mg/kg) | Lead acetate (50 mg/kg.b.w.) + Cranberry extract (150 mg/kg) |
(Hepatic tissue) | ||||
GSH (nmol/g. tissue) |
2.80 ± 0.032a |
0.78 ± 0.022d |
1.28 ± 0.097c |
1.94 ± 0.079b |
SOD (U/g. tissue) | 3.70 ± 0.06a |
1.09 ± 0.078d |
1.94 ± 0.025c |
2.81 ± 0.047b |
CAT (U/g. tissue) |
1.82 ± 0.030a |
0.77 ± 0.026d |
1.13 ± 0.046c |
1.45 ± 0.032b |
MDA (mmol/g. tissue) |
16.22 ± 0.27d |
40.96 ± 0.51a |
28.36 ± 0.73b |
20.18 ± 0.32c |
TAC (mM/g. tissue) |
1.26 ± 0.030a |
0.58 ± 0.026d |
0.87 ± 0.015c |
1.08 ± 0.024b |
(Renal tissue) | ||||
GSH (nmol/g. tissue) |
2.14 ± 0.067a |
0.66 ± 0.019d |
1.06 ± 0.083c |
1.75 ± 0.055b |
SOD (U/g. tissue) |
3.07 ± 0.032a |
1.94 ± 0.059c |
2.39 ± 0.161b |
2.89 ± 0.018a |
CAT (U/g. tissue) |
1.27 ± 0.067a |
0.64 ± 0.022d |
0.84 ± 0.016c |
1.06 ± 0.049b |
MDA (mmol/g. tissue) |
12.02 ± 0.48d |
32.80 ± 1.13a |
21.26 ± 0.70b |
16.42 ± 0.35c |
TAC (mM/g. tissue) |
1.10 ± 0.037a |
0.66 ± 0.034c |
0.75 ± 0.018c |
0.89 ± 0.024b |
abcd Means with different superscript within same row are statistically different at level P<0.05 according to Duncan’s multiple range test.
Table 7: Histological lesion score among different experimental groups of rats.
Groups lesion | Normal group | Positive control (lead acetate 50 mg/kg.b.w.) | Lead acetate (50 mg/kg.b.w.) + Cranberry extract (75 mg/kg) | Lead acetate (50 mg/kg.b.w.) + Cranberry extract (150 mg/kg) |
(Liver) | ||||
Congested blood vessels and sinusoids | - | +++ | ++ | ++ |
Degenerated hepatocytes | - | ++++ | +++ | + |
Inflammatory cells aggregations | - | ++++ | - | - |
Portal fibrosis | - | ++ | - | - |
Kupffer cells hyperplasia | - | - | ++ | + |
Diplocytes (Regenerative attampts) | - | - | + | +++ |
(Kidney) | ||||
Necrotic glomeruli | - | ++ | - | - |
Shrunken/lobulated glomeruli | - | +++ | +++ | - |
Tubular casts | - | +++ | + | - |
Hemorrhages | - | ++++ | - | - |
Histological lesions Score system (-: No Alterations 0%; +: Mild Alterations 10-35%; ++: Moderate Alterations 40-50%; +++: Severe and ++++: Intense Alterations up to 60%.
Histopathological findings of liver and kidney tissue in different groups of rats
Table 7 and Figure 1 Representative Photomicrograph of rats liver (H and E, X400) demonstrating: (A) Structures of normal hepatic tissue. (B) Inflammatory accumulations replace necrotic liver cells (star). (C) Interlobular fibrotic strands (star). (D) Portal fibrosis besides congested blood vessels and proliferated bile duct epithelium (star), (E) Restore normal hepatocytes and hyperplastic kupffer cells with a few hepatocytes appeared apoptosis (arrow). (F) Apparently normal hepatocytes with prominent diplocytes (arrow) with still slight congested blood vessels. A) Control, B, C and D) Lead toxicity, E) Lead + Cranberry (75mg), Lead + Cranberry (150mg) n=10.
Table 7 and Figure 2 representative photomicrograph of rats kidney (H and E, X400) demonstrating: (A) Structures of normal renal tissue. (B) Renal tubules with severe necrosis and glomeruli (arrow) besides edema infiltrated with inflammatory cells (star). (C) Degenerated renal tubules, interstitial inflammatory cells infiltrations, extravasated erythrocytes (star) and vasculitis characterized by endotheliosis and vacuolar media (arrow). (D) Prominent homogenous more eosinophilic cats in the convoluted renal tubules (arrow), (E) remodeling the majority renal structures with regenerated attapts (arrow). (F) Apparently normal renal glomeruli and proximal tubules. A) Control, B, C and D) Lead toxicity, E) Lead + Cranberry (75mg), Lead + Cranberry (150mg) n=10.
There is various type of heavy metals within the ecosystem (waters, soil and dust) and this cannot be degraded. The key sources of heavy metals are various forms of anthropogenic practices including mining, melting and various types of industrial trash (Wu et al., 2015). Earth ‘s crust usually contains heavy metals in trace quantities, however in several aspects of everyday lives are they used, for example, Putters, self-washing ovens, vehicles, disinfectants, plastics, phones, sunlight power plates, fuel cells, as well as several other things (Karthik et al., 2016).
Heavy metals that count lead constitute a significant health hazard to living creatures (Abou-Kassem et al., 2016; Khafaga, 2017; Saad et al., 2018). Throughout most aspects of nature, lead as well as other heavy metals are noticeable in conjunction with many other elements at very small pollution levels; thus, the existence of lead in the ecosystem does not perform any function in physiological systems. It might result in permanent health problems with a high rate of morbidity, like hepatic, nervous, and renal system diseases (Murata et al., 2009; Ekanem et al., 2015). Extreme lead poisoning causes problems with body function, including neurological, cardiovascular, hematologic, and reproductive issues. Elevated blood lead concentrations trigger the central nervous system (CNS) to malfunction, resulting in encephalopathy and edoema, which directly targets the cerebellum (Rao et al., 2014; Pal et al., 2015). Pregnant women who have an elevated concentrations of lead in their bodies may have abortion. Males’ potency was reduced when they were exposed to lead for a long time (Vigeh et al., 2011; Amadi et al., 2017).
Use of phytonutrients in the last years to protect against the dangers of environmental toxic substances has been viewed as a promising pharmacological strategy to maintain health of the body without side effects (Kumar et al., 2009). According to Vinson et al. (2001), cranberries’ antioxidant properties are unique among fruits in terms of quality and quantity of antioxidants because they contain a high concentration of flavonoids and phenolic acids. Therefore, the goal of this work aimed to investigate if cranberry extract could protect rats from hepato-renal toxicity caused by lead acetate.
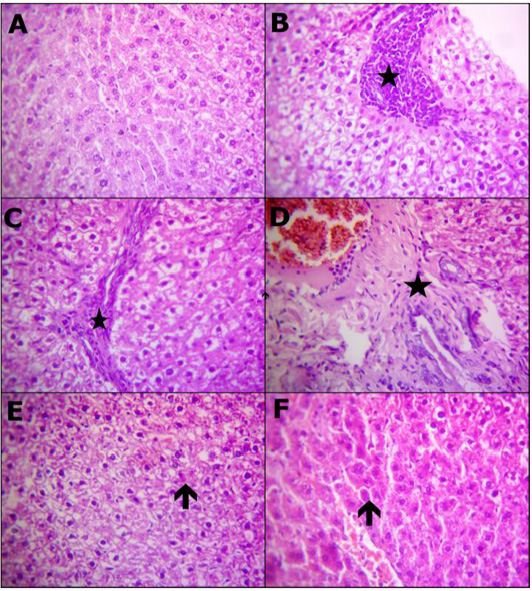
Figure 1: Reprehensive photomicrograph of rats liver (H and E, X400) showing; (A) Normal hepatic micromorphological structures; (B) Necrosis hepatocytes replaced by inflammatory aggregations (star); (C) Interlobular fibrotic strands (star); (D) Portal fibrosis besides congested blood vessels and proliferated bile duct epithelium (star); (E) Restore normal hepatocytes and heperplastic Kupffer cells with a few hepatocytes appeared apoptosis (arrow); (F) Apparently normal hepatocytes with prominent diplocytes (arrow) with still slight congested blood vessels. (A) Control; (B, C and D) Lead toxicity; (E) Lead + Cranberry (75mg), Lead + Cranberry (150mg) n=10.
GC/MS results of cranberry extract revealed that major identified compounds were 29.33% of stearic acid and 10.44% Palmitic acid; major constituents identified in extract were displayed in (Table 1). Our findings are consistent with some previous research, which identified stearic and palmitic acids as the major components of the cranberry extract’s chemical composition (Pappas and Schaich, 2009; Glisan et al., 2016).
When compared to the normal group, the lead acetate-caused liver damage markedly raised serum liver enzymatic activity (ALT, AST, LDH, and ALP), also total soluble serum protein, albumin and globulin concentrations were reduced significantly, as well as a large rates of liver histologic alterations. Increased hepatic enzymes have been utilized as assays of hepatic injury. They enter bloodstream as a result of lipid oxidation caused by hepatic membrane stability loss induced by lead poisoning (Farida et al., 2012; Laamech et al., 2017). Since plasma proteins in the liver, particularly albumin, are mainly synthesized, a substantial decrease of total protein in serum indicated liver dysfunction (Abdel-Maksoud et al., 2015a). Furthermore, because lead binds to plasma proteins, it disrupts protein synthesis in hepatocytes, which leads to variations among large amount of enzymes of hepatocytes (Shalan et al., 2005). It moreover disrupts the signal of Ca2+ inside the cells, leading to endoplasmic reticulum’s demolition and reducing free amino acids application to synthesize proteins (Moussa and Bashandy, 2008; BaSalamah et al., 2018). Comparable findings were observed in rats given sub-lethal doses of lead acetate (1/20, 1/40, 1/60) by Ibrahim et al. (2012). Similar results in lead acetate-intoxicated rats have been observed by El-Tantawy (2016) as well as Gargouri et al. (2017).
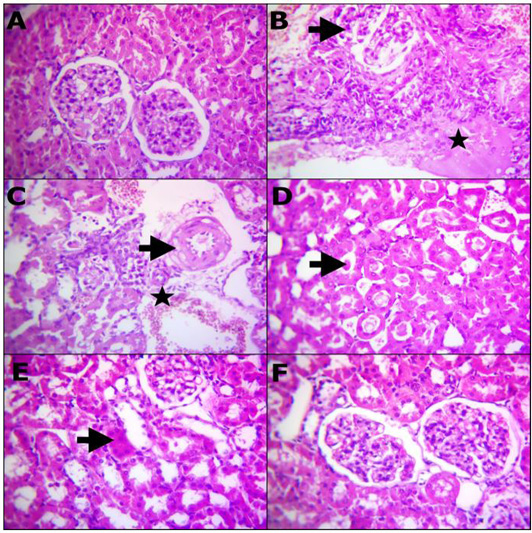
Figure 2: Reprehensive photomicrograph of rats Kidney (H and E, X400) showing; (A) Normal renal histomorphological structures; (B) Massive necrotic renal tubules and glomeruli (arrow) besides edema infiltrated with inflammatory cells (star); (C) Degenerated renal tubules, interstitial inflammatory cells infiltrarions, extravasated erythrocytes (star) and vasculitis characterized by endotheliosis and vacuolar media (arrow); (D) Prominent homogenous more eosinophilic cats in the convoluted renal tubules (arrow); (E) Remodeling the majority renal structures with regenerated attapts (arrow); (F) Apparently normal renal glomeruli and proximal tubules. (A) Control; (B, C and D) Lead toxicity; (E) Lead + Cranberry (75mg), Lead + Cranberry (150mg) n=10.
In comparison to the group that received lead acetate, addition of 75 and 150 mg/kg cranberry extract led to a marked reduction in serum alanine aminotransferase (ALT), aspartate aminotransferase (AST), lactate dehydrogenase (LDH), total bilirubin, direct bilirubin, alkaline phosphatase (ALP) levels as well as a marked rise in serum total soluble protein, albumin and globulin levels.
The current investigation found increased serum ALT, AST, LDH, and ALP (a marker of hepatocyte damage) activities as a result of oxidative stress caused by Pb+2. These enhanced activities could be linked to the spilling of these enzymes into bloodstream from damaged hepatocytes due to altered membrane permeability of hepatocytes (Shohda et al., 2009). Increased serum ALP activities indicate cellular damage caused by a loss of cell membrane functional integrity. Hesperidin effectively reduced the blood accumulation of Pb+2 and showed that the polyphenols of cranberries chelate Pb+2. Furthermore, polyphenolic hydroxyl groups or their active metabolites could bind with Pb+2 and increase its excretion, lowering Pb+2 accumulation and reducing its toxicity. Hesperidin, a cranberry flavonoid, is well known for its antioxidant properties (Chanet et al., 2012), can remove excess Pb+2 from biological systems. Lipid metabolism modifications were triggered by Pb+2 and also serum and tissue lipids levels alterations. It is possible because Pb+2 in the liver has accumulated, which is important for lipid homeostasis. Excess Pb+2 causes cellular damage and functional hepatocyte abnormalities through lipid peroxidation (Britton et al., 1987). Since the liver plays such an important role in maintaining lipid homeostasis, excess Pb+2 can alter serum lipid concentration, which can reduce or increase atherosclerosis risk.
In preclinical studies, serum creatinine and urea are proposed for assessing kidney injury because they are regarded the most specified and susceptible markers of renal failure. Serum creatinine and urea concentrations are often low in the bloodstream, however they rise once the renal become ill or injured. Most rises in serum creatinine and urea are due to renal injury (Moussa and Bashandy, 2008). This study demonstrated that rats fed lead acetate had higher concentrations of creatinine and urea leading to renal failure as compared to the normal control group. Ghoniem et al. (2012) made a same result, reporting that the treatment with lead acetate resulted in a considerable rise in serum creatinine and urea levels. In comparison to the group receiving lead acetate, intake of cranberry extract at a dose of 75 and 150 mg/kg led to a marked reduction of serum levels of creatinine and urea, showing that it would have a protective action of the kidneys versus renal toxicity caused by lead acetate. It could be because of its effective activity on lead acetate reactive oxygen species, which safeguards the kidney from tissue destruction through preserving membrane safety.
The significantly higher concentrations of total cholesterol and triglyceride of the lead acetate group had been caused whether by accelerated formation or reduced clearance, since lead is believed to trigger a lipoprotein cell surface receptor malfunction or restricted the enzymatic action of hepatic lipoprotein lipase (Liu et al., 2011). Pb+2 also decreased the cytochrome P-450 action which is crucial to bile acid biosynthetic pathway, that is the primary pathway in the body in order to remove cholesterol (Newairy and Abdou, 2009; Dewanjee et al., 2013). Offor et al. (2017) have reported similar results.
Peroxidation of lipid might still destroy various cell membranes, causing changes in blood pressures as well as regulation of the heartbeat. Considering the fact that LDL cholesterol (LDL-C) levels are higher as well as HDL cholesterol levels are lower (HDL-C). Overloading iron caused an increase in total cholesterol as well as triglycerides, as previously reported by Dabbagh et al. (1994) and Brunet et al. (1999). The Fe+3 produced an increase in serum or tissue cholesterol was often caused by alterations of liver enzymes gene expression, primarily HMG-COA reductase. This HMG-COA enzyme genomic alteration induced by heavy metals were reported as well (Kojima et al., 2004). In Fe poisoned rats, increased plasma lipids (PLs) could be due to increased FFAs and cholesterol levels. Cranberry polyphenols reduced the unusual scattering of membrane lipids in circulatory system and the elevated production of further harmful peroxides, that induce severe alterations in tissues and cells, possibly due to their antioxidant properties. Howard and Kritchevsky (1997) clarified that decreased cardio-vascular risk is generally associated with phytochemicals that reduce the level of high cholesterol as well as triglycerides consumption.
In comparison with normal control group, oral intake of lead acetate at a dose of 50 mg/kg led to a substantial rise in serum glucose and tumor necrosis factor-alpha (TNF-α) levels as well as a significant reduction in serum triiodothyronine (T3) and total thyroxine (T4) levels.
The pro-inflammatory cytokines in the liver (TNF-α, IL-1β, and IL6) were significantly upregulated after LA administration. Both BaSalamah et al. (2018) and Liu et al. (2018) reported that lead exposure promotes NF-κB activation and enhanced inhibiting phosphorylated adenosine monophosphate-activated protein kinase (AMPK), that subsequently increases reactive oxygen species and inflammatory cytokines in serum and tissues. These findings match those of El-Tantawy (2016), who found a substantial elevation in the values of tumor necrosis factor-alpha as well as caspase-3 in the liver of rats exposed to lead acetate poisoning at a dose of 100 mg/kg each day up to four weeks. Likewise, Gargouri et al. (2017) and El-Boshy et al. (2019) observed that rats exposed to lead acetate toxicity had greater levels of IL-1β, TNF-α, and IL-6 in serum.
In comparison to the group that received lead acetate, addition of 75 and 150 mg/kg cranberry extract led to a marked decrease in serum glucose and tumor necrosis factor-alpha (TNF-α) levels as well as a marked rise in serum triiodothyronine (T3) and total thyroxine (T4) levels.
Following that, several genetic expressions such as cyclooxygenase-2 (COX-2) as well as inducible nitric oxide synthase (iNOS) were modulated, resulting in a reduction in tumor necrosis factor-alpha (TNF-α) as well as Interleukin 1 beta (IL-1β) production according to Hosseini and Hosseinzadeh (2018). These findings agree with those reported by Fu et al. (2008), and El-Sherbiny et al. (2010), as well as Ali et al. (2018), in which the pro-inflammatory serum TNF-α was decreased by cranberry extract.
In comparison with normal group, oral intake of lead acetate at a dose of 50 mg/kg led to a marked reduction in reduced glutathione (GSH) level, superoxide dismutase (SOD), catalase activity (CAT) and total antioxidant capacity (TAC) of hepatic and renal tissues. Also, the level of malondialdehyde (MDA) has increased significantly in hepatic and renal tissues.
Lead poisoning has a mechanistic and major role in the occurrence of oxidative stress possibly through increasing reactive oxygen species production and even exhausting the anti-oxidative enzymes strategy, as previously reported by El-Tantawy (2016); Laamech et al. (2017) and El-Boshy et al. (2019). Moreover, Pb+2 attaches permanently to the thiol group of GSH and is eliminated into the bile according to Ercal et al. (2001) and El-Tantawy (2016). Moreover, it attaches toward additional SH-having proteins, such as superoxide dismutase and catalase activity, lowering its function leading to increased lipid peroxidation as well as degradation of DNA, as previously reported by Andjelkovic et al. (2019). During this present investigation, rats subjected to lead acetate had a large increase in MDA values in both homogenized liver and kidney, as well as a substantial decrease in superoxide dismutase and catalase activity, and also a substantial drop in glutathione values. These findings were in agreement with BaSalamah et al. (2018). He found a large elevation in the values of malondialdehyde of liver and kidney, as well as a substantial drop in Glutathione Peroxidase and catalase activity, whereas superoxide dismutase was unaffected. Hepatic MDA was markedly increased in this context, while the levels of reduced glutathione as well as superoxide dismutase in the rats exposed to lead acetate toxicity were statistically decreased according to Baxla et al. (2013). In addition, Laamech et al. (2017) demonstrated that lead-exposed mice showed a marked decrease in the anti-oxidative enzymes of the liver including CAT, SOD, GPx, and GSH as well as a rise in MDA levels. These results are in agreement with those reported by El-Sokkary et al. (2005); Mehana et al. (2012); Dewanjee et al. (2013) and Gargouri et al. (2017) as well.
In the present study, cranberry extract co-treatment at 75 and 150 mg/kg led to a marked increase in the levels of reduced glutathione (GSH), superoxide dismutase (SOD), catalase activity (CAT), as well as total antioxidant capacity (TAC) in the tissues of liver and kidney, also a marked reduction in malondialdehyde (MDA) levels in comparison to lead-acetate group, which shows a large enhancement in blood profile changes according to El-Maddawy and El-Sayed (2018). Moreover, liver injury was slightly lowered by CBE, which had been histologically indicated, as well as linked to limited improvement in liver enzymes, returned standard serum total protein, albumin, and globulin values, as well as decreased lipid peroxidation, as evidenced by lower MDA concentrations in the liver, as well as repaired superoxide dismutase and catalase activity. These findings possessed the capability of cranberry extract in maintaining cellular membranes as well as safeguarding tissues against destruction by free radicals, as well as enhancing the regeneration of damaged cells and hence decreasing transaminase and ALP release from the cytosol as previously reported by Abdel-Daim and Abdou (2015), El-Maddawy and El-Sayed (2018), Cheraghi and Roshanaei (2019) as well.
In comparison to control rats, the histopathological results demonstrated reversible degrading impacts affecting hepato-renal formation of rats subjected to lead-acetate toxicity, which could have been attributable to increased oxidative damage (Figure 1B-1D) and (Figure 2B-2D). These degenerative changes have been observed on female albino rats following exposure to lead and because of oxidative damage as well as hepato-renal oxygen free radicals (Ghoniem et al., 2012). This investigation has demonstrated that lead acetate administration of lead-acetate resulted in several hapatic histological changes, including PV obstruction, Kupffer cell hyperplasia, the inflammatory cell central region, blood sinusoidal contraction, and hepatocyte binucleation many histological changes in the liver, including PV congestion, Kupffer cell hyperplasia, focal area of inflammatory cells, contraction of blood sinusoids, as well as double nucleus formation of liver cells. Dehkordi et al. (2015); Omotoso et al. (2015) have reported similar results. The contact between Pb+2 and enzymes as well as tissue proteins involved in the liver, that mostly interacts with the anti-oxidative system and leads towards oxygen free radicals production, could cause hepatic inflammatory cells formation upon toxicity caused by lead-acetate. These could be used to simulate the reactions that cause inflammation according to Johar et al. (2004). Binucleation, as seen in this study, could be a response to cellular damage that typically shown through renewable cells (Gerlyng et al., 1993). Lead stimulates the phagocytic action of sinusoidal cells by increasing stellate sinusoidal macrophages in response to autophagy elevation often throughout liver tissue, which aids throughout eliminating removal of excessive Pb+2 as well as accompanying metabolites, according to findings of this study. The resulting hyperplasia of Kupffer cells is a detoxification defense technique (Ilić et al., 2014). Histopathological examinations of the liver reveal changes that indicate hepatic tissue destruction probably as a result of heavy metal cycling. Gajawat et al. (2005) clarified that toxic metal generate mercaptides containing cysteine’s sulfhydryl group as well as weaker steady compounds among several chains of amino acids, which indicate hepatic tissue destruction.
Throughout comparing with Pb+2 rats treated group, Figure 1E revealed the big importance of using cranberry extract, which showed a significant improvement in histological structure of rats hepatic tissues as well as fewer pathogenic alterations. Cranberry extract has been shown to have identical improvements actions occurring in the rats hepatic tissues upon alterations caused by lead-acetate toxicity (Koriem, 2009).
Lead acetate administration resulted in glomerular changes, according to our histological examination of renal tissue. These changes included glomeruli atrophy and tubular epithelial cell degradation, which was consistent with previous research (Abdou and Hassan, 2014; Sharma and Singh, 2014). The cytoplasmic degradation noticed could be due to lysosomal hydrolytic enzyme leakage (Del Monte, 2005).
Cranberry extract has been shown to preserve histological integrity in destroyed liver and renal tissue with parenchyma necrosis, tubular expansion, and hyperemic conditions (Abdel-Maksoud et al., 2015b). The use of cranberry extract to lead-treated rats enhanced the structure and partially restored the investigated parameters to standard levels, as well as reducing the histopathological modifications rigidity (Figure 2E). Our observations appeared familiar to that by Abdel-Maksoud et al. (2015c) publications, showing similar interference impacts of excess iron in experimental rats.
Paracetamol-treated rats for hepatic tissues were found to have normal histoarchitecture after the use of cranberry extract, according to Abdel-Maksoud et al. (2015b). The existence of flavonoids was discovered during a phytochemical preliminary examination of cranberry extract. Flavonoids (or bioflavonoids) are natural components that can modulate the enzymatic activity of catalase and superoxide dismutase as well as influence multiple cellular systems’ behaviors. They also have anti-inflammatory, antihepatotoxic, antitumor, anti-allergic, anti-osteoporosis and antioxidant properties (Ilić et al., 2014; Abdel-Maksoud et al., 2015a).
Conclusions And Recommendations
In conclusion, the present study demonstrates that cranberry supplementation has protective effects against hepato-renal toxicity caused by lead acetate in rats. This could result from its antioxidant action, which includes the power of chelating ROS as well as prevent peroxidation of lipids, that can often cause cellular injury in the hepato-renal system. Furthermore, this could be a key toward the development of new free radical products that are both safe and effective.
Novelty Statement
This study discovers the protective activity of cranberry extract that can be beneficial for the treatment of lead acetate-induced liver and renal toxicity. This study will help the researcher to uncover the critical areas that focus on evaluating of cranberry extract as a promising new agent in the treatment of certain type of liver and renal inflammation that many researchers were not able to explore. Thus, a new theory to explain the correlation between protective activity of cranberry extract and the level of antioxidant enzymes in liver and renal tissue may be arrived at.
Author’s Contribution
Experimental design, antioxidant and hepato-renal-protective activities of cranberry extract were carried out by Hussein Ibrahim El-belbasy, Mohammed Abdalla Hussein, Mohamed El-mitwaly Alghitany. Wrote the protocol, wrote the first draft of the manuscript, managed the analyses of the study, managed the literature searches was carried out in collaboration between all authors.
Conflict of interest
The authors have declared no conflict of interest.
REFERENCES