Advances in Animal and Veterinary Sciences
Research Article
Advances in Animal and Veterinary Sciences 2 (4): 248 – 254Persistence of Highly Pathogenic Avian Influenza Virus (H5N1) in Soil Samples at Different Temperatures
Huchhegowda K.H*, Murugkar H.V.1, Nagaraja K.H.1, Manoj Kumar1, Nagarajan S.N.1, Tosh C1 , Ashok Kumar1
- High Security Animal Disease Laboratory, IVRI, Anand Nagar, Bhopal – 462012
*Corresponding author: [email protected]
ARTICLE CITATION:
Huchhegowda KH, Murugkar HV, Nagaraja KH, Kumar M, Nagarajan, SN, Tosh C, Kumar A (2014). Persistence of highly pathogenic avian influenza virus (H5N1) in soil samples at different temperatures. Adv. Anim. Vet. Sci. 2 (4): 248 – 254.
Received: 2014–03–08, Revised: 2014–04–02, Accepted: 2014–04–03
The electronic version of this article is the complete one and can be found online at
(
http://dx.doi.org/10.14737/journal.aavs/2014/2.4.248.254
)
which permits unrestricted use, distribution, and reproduction in any medium, provided the original work is properly cited
ABSTRACT
Avian Influenza caused by H5N1 is one of the most important zoonotic diseases and is becoming a great threat to poultry industry as well as to humans. Although wild aquatic birds are the main reservoir for avian influenza viruses, the environment plays a critical role for the circulation and persistence of avian influenza virus. Contaminated water and soil may play roles as reservoirs and sources of transmission for avian influenza virus. However, very little is known regarding the persistence of highly pathogenic avian influenza (HPAI) H5N1 viruses in aquatic environments and soil near the water bodies in tropical countries. The aim of this work was to study the persistence of HPAI (H5N1) virus in soil samples at different temperatures and the physicochemical features of the sources. Soil samples were collected from three different lakes and two ponds in Madhya Pradesh, India and two important physico–chemical parameters (soil moisture and soil pH) were estimated for soil samples. HPAI virus (H5N1) isolate (A/Chicken/Manipur/India/59001/07) was spiked in soil samples and incubated at two different temperatures (12ºC and 25ºC). The samples were processed every 24 hrs for the isolation of virus in 9 – 11 day old chicken embryos and for detection of viral RNA by carrying out Real Time Reverse Transcriptase PCR (qRT–PCR). Mean persistence of HPAI virus in soil samples at 25°C and 12°C were 8 days and 15.4 days with ranges from 1–18 days and 5 to 29 days, respectively. Mean persistence of viral RNA in soil samples at 25°C and 12°C was 9.6 days and 16.6 days, respectively. HPAI virus (H5N1) persisted longer at 12°C as compare to 25°C indicating a strong negative correlation between the survivability of the virus and temperature. Soil pH showed a strong positive correlation at both the temperatures and moisture content showed strong negative and moderate negative correlation on viral persistence at 12°C and 25°C, respectively The study gives insights into the various physicochemical factors involved in the persistence of avian influenza virus in soil and water and should play an important role in formulating avian influenza control strategies.
INTRODUCTION
Avian influenza (AI) is a notifiable disease caused by type A influenza virus and is a member of the Orthomyxoviridae family. AI viruses are responsible for major disease problems in birds, as well as in mammals including humans and the disease are mainly transmitted by aerosol and faeco–oral route. The environmental persistence of virus depends on many factors such as, local environmental conditions, viz., temperature, salinity and organic matter (faeces) (Brown et al., 2007). Several reports suggest that environment may be contaminated from respiratory secretions and faeces and may remain infective at ambient temperatures for weeks or months, even longer in the refrigeration conditions (Stallknecht et al., 1990). Many experiments have reported the routine isolation of many AIVs subtypes, including H5, from unconcentrated surface water (Hinshaw et al., 1979), mud and soil swabs and from aquatic environments where previous outbreaks were documented (Liu et al., 2004). Low temperatures are also found to increase the stability of the viruses. It was also reported that the virus is capable of surviving on contaminated surfaces such as the poultry house for several weeks. The duration of viral infectivity is related to both the concentration and the presence of a beneficial microenvironment. Previous studies show that the low pathogenic influenza viruses remain infectious in lake water for more than 30 days at 0⁰C and for up to 4 days at 22⁰C (Webster et al., 1978). Recent studies revealed that H5 and H7 avian influenza viruses, including highly pathogenic strains, have the ability to persist in water with wide variety of temperature and salinity for extended periods of time (Brown et al., 2007). The longstanding evolutionary and ecological relationship between wild birds and AIV has created a broad pool of viral genetic diversity and a reservoir of potentially transmissible viruses. The pond, river or lake water plays an important role in this mechanism. However seasonal variation in different countries or parts of a country may be a critical factor for consideration. It has been generally accepted that fecal–oral transmission of AIV, particularly within wild waterfowl populations is thought to occur via contaminated water, however, very little is known about its persistence in this medium under natural state. The persistence of virus in the environment may be a reason of repeated outbreaks and due to lack of virus specific information on the presence and stability of the H5N1 virus in environment, it is very important to study on this issue comprehensively. The survivability of the virus under different seasons in organic matter and water bodies has not been studied in India. Therefore this project was proposed with the objective to study the persistence of Highly Pathogenic Avian Influenza Virus (H5N1) in soil samples at different temperatures.
MATERIALS AND METHODS
Sample Collection
In the present study soil samples were collected from the banks of the three lakes and two ponds in Madhya Pradesh, India. All soil samples were transported at ambient temperature (25–27ºC) from the collection site to the laboratory within 1 hour and stored at 4ºC. These samples were used to conduct the experiments within 24 hrs after field collection. The absence of virus in all soil samples was verified by qRT–PCR prior to use for the experiments. In order to rule out the presence of any inherent toxicity or infective agent in the soil samples that may kill the embryo were processed for egg inoculation prior to use for the experiments. The eggs were incubated at 37⁰C for five days and mortality, if any was observed in the eggs.
Estimation of Physico–Chemical Parameters
Two important physico–chemical parameters were estimated for each soil samples viz. pH, moisture content with the help of standard protocol.
Estimation of pH
The pH meter was calibrated by dipping the electrode in standard phosphate buffers of 7.0, 4.0 and 9.0 pH range and the electrode was cleaned by dipping them into triple distilled water and blot dried. 10 g of sieved, air–dried soil samples were transferred into the beakers. 10 ml of distilled water was added to all the beakers containing soil samples from different sources. Soil samples were mixed thoroughly with the distilled water to get slurry and kept at room temperature for 30 minutes. Placed the electrode in the slurry for 1 minute and pH was noted down (McLean, 1982).
Estimation of Moisture Content
Thirty grams of representative moist soil samples were transferred to the pre–weighed sterile beakers and weight of the beakers with soil samples were noted down. Beakers were then incubated at 105°C in a hot air oven for 24 hrs. The beakers were allowed to cool in a desiccator for 1 hr. After cooling, the beakers were again weighed and final weight was noted. The moisture content of the soil samples was calculated by using the following formula (Standards Association of Australia. AS 1289 B1.1, 1977).
Moisture content (%) = ((W2) – (W3) /(W3) – (W1)) x 100
Where,
• W1 – Beaker weight
• W2 – Moist soil weight + Beaker weight
• W3 – Dry soil weight + Beaker weight
Spiking of Optimum Concentration of Virus in Soil Samples
Virus with known EID50 was serially diluted (10–fold) and 50 μl virus of each dilution was spiked to 5 g of soil samples and incubated at room temperature for 1hr. Soil samples were processed by using elution and concentration method (as mentioned below) and isolation of virus was carried out in 9 – 11 day old embryonated chicken eggs (after processing the pellet suspension in 1X PBS from each dilution was inoculated to 3 ECEs). Eggs were incubated at 37°C for 5 days, harvested and HA has done. The infective titer for this experiment was standardized following serial tenfold dilution of the standard virus and 104 EID50 virus/gram of the soil sample was estimated to be the optimum infective dose for the experiment. Five grams of soil from each source was aliquoted in 50 ml centrifuge tubes and accordingly, 50 μl of 106 EID50 virus per ml of the allantoic fluid was used to spike each 5 g aliquot. After spiking soil samples were incubated at 12ºC and 25ºC and were tested in every 24 hrs interval.
Isolation of Virus
The isolation from the spiked soil samples was done by elution and concentration method (Horm et al., 2011). Twenty millilitre of 10 % beef extract elution buffer (pH 7) were added to the centrifuge tubes containing soil samples. The tubes were then placed on a magnetic stirrer and stirred for 30 min at room temperature at a speed sufficient to form a vortex. The tubes were then centrifuged at 7,000 g for 30 min at 4ºC. The supernatants were collected and the sediment was discarded. Equal volume of 16 % PEG 6000 solution was gently added to each eluate sample and stirred at a very low speed. The mixtures were incubated at 4ºC for 2 hrs and then centrifuged at 7,000 g for 1 hr at 4ºC. The pellets were recovered and dissolved in 1.2 ml of PBS and 200 μl of this suspension was transferred to sterile microcentrifuge tube for RNA detection by using qRT–PCR. To the remaining suspension, 10% antibiotic and antimycotic mixture was added. The mixture was pipetted into a microcentrifuge tube and incubated for 1 hr at 37ºC. Tubes were mixed by inverting three or four times during the incubation period.
The processed sample was aspirated into a syringe with a 22 gauge, 1 ½ inch needle. The needle was inoculated at 45 degree angle from the hole with a short stabbing motion and the entire volume was used to inoculate five 9 – 10 day–old embryonated chicken eggs with equal volumes of the mixture by amnio–allantoic cavity route. The eggs were incubated at 37°C for up to 5 days or till death of the embryo which ever was earlier. The embryos were candled every day at 12 hr interval. All the dead embryos including those dead within 24 hr were chilled at 4°C. After 5 days, all the live embryos were removed from the incubator and chilled at 4°C for 24 hr (WHO, 2002). The amnio–allantoic fluid from each egg was collected separately at room temperature in a class IIB biosafety cabinet with appropriate personal protection clothing. The harvested fluids were centrifuged at 8,000 rpm for 5 min to remove blood and cells. The clear supernatant was aseptically transferred into sterile tubes and hemagglutination test (OIE, 2012) was performed to confirm the presence of the virus. Molecular Confirmation of Viral RNA
QIAamp viral RNA mini Kit (Qiagen, Germany) was used to extract viral RNA from the inoculums which were stored at –80°C as per the manufacturer’s protocol. Real Time RT–PCR system, LightCycler® 480 Real–Time PCR (Roche, USA) was used. The primers (H5 specific) and probe (H5 specific) for amplification of HA gene were used as shown in the Table 1. Real time RT–PCR reaction mixture was prepared and all the tubes were kept in Real time PCR machine and then the program was started.
Statistical Analysis
The persistence of virus was determined by calculating the percent infectivity as described by (Baleshwari, 2010). The formula used to calculate the Percent infectivity is as follow:
% Infectivity = (Infected embryos (No. of HA positive) / Total embryos inoculated) x 100
The variables considered for the statistical analysis consisted of percent infectivity as dependent variable and time as the independent variable. The effect of different temperatures on the persistence of H5N1 was determined by linear regression model and defined in terms of linear equation. Coefficient of determination (R2) indicated the goodness of fit of a model and explains that how well the regression model approximates the real data points (Microsoft excel 2007). Coefficient of correlation (r) was calculated to study the effect of physico–chemical parameters of soil samples on the viral persistence at 12ºC and 25ºC (Microsoft excel 2007). The strength of the correlation was estimated by using the guidelines given by the Cohen (1988). Critical values for the Pearson correlation was used to know whether the estimated coefficient of correlation (r) for each parameter is significant or not (Fisher and Yates, 1974). In the present study sample size used was 5 so degrees of freedom are 3. For samples of size 5 (df = 3), the critical value is 0.805 for the 0.1 level of significance, 0.878 for the 0.05 level and 0.959 for the 0.01 level of significance. To say Pearson ‘r’ is significant, it should equals or surpasses the critical value at desired level of significance (α). In present level of significance (α) used was 0.05.
RESULTS
Two physico–chemical parameters of the soil samples were estimated in the laboratory as per the standard protocols and listed in Table 2. The infective titer for this experiment was standardized following serial tenfold dilution of the standard virus and 104 EID50 virus/gram of the soil sample was estimated to be the optimum infective dose for the experiment. Accordingly, 50 μl of 106 EID50 virus per ml of the allantoic fluid was used to spike each 5 g aliquot.
Persistence of HPAI Virus (H5N1) in Soil Samples at 12°C
A set of 50 centrifuge tubes of each soil samples was prepared and kept at 12°C and the persistence of HPAI virus (H5N1) at 12°C was detected every 24 hrs. In the first passage the virus was isolated up to 5, 29, 13, 16 and 14 days post spiking in Lake 1, Lake 2, Lake 3, Pond 1 and Pond 2 soil samples, respectively (Figure 1). In second and third passage also virus could not be isolated from the soil samples found negative in first passage. The viral RNA was detected by qRT–PCR up to 5, 31, 13, 18 and 16 days post spiking in Lake 1, Lake 2, Lake 3, Pond 1 and Pond 2 soil samples, respectively (Fig. 1 and 2). Viral RNA could not be detected in Lake 1 soil on 6 and 7 DPS, in lake 2 soil on 32 and 33 DPS, in lake 3 soil on 14 and 15 DPS, in Pond 1 soil on 19 and 20 DPS and in Pond 2 soil on 17 and 18 DPS samples (Figure 2). The linear regression analysis indicated that the expected time required for virus to become undetectable was 7.42, 34.55, 16.59, 22.23 and 15.71 days against the observed values of 6, 30, 14, 17 and 15 days in Lake 1, Lake 2, Lake 3, Pond 1 and Pond 2 soil samples respectively. The coefficients of determination (R2) values for Lake 1, Lake 2, Lake 3, Pond 1 and Pond 2 soil samples were estimated to be 0.815, 0.866, 0.887, 0.807 and 0.948 respectively (Table 3 and Figure 3a–e).
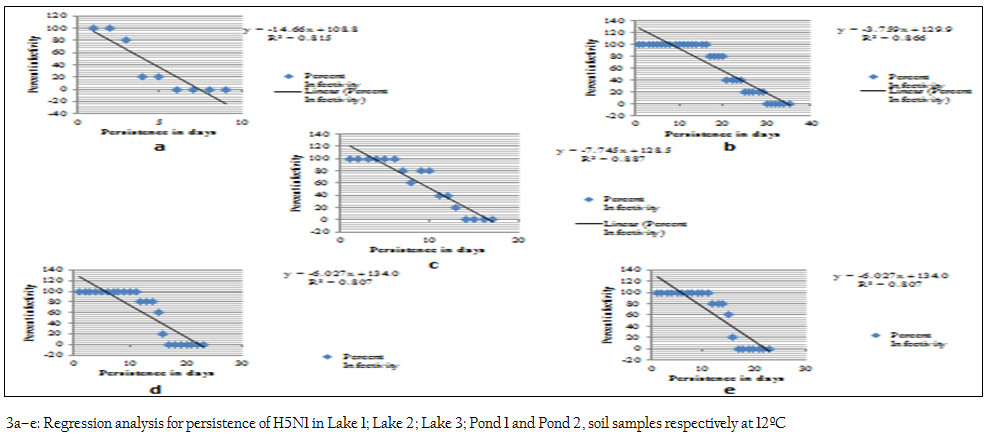
Figure 3: a–e: Regression analysis for persistence of H5N1 in Lake 1; Lake 2; Lake 3; Pond 1 and Pond 2, soil samples respectively at 12ºC
Persistence of HPAI Virus (H5N1) in Soil Samples at 25°C
A set of 35 centrifuge tubes of each soil sample was prepared and kept at 25°C and the persistence of HPAI virus (H5N1) at 25°C was detected every 24 hrs. In the first passage the virus was isolated only up to 1, 18, 7, 5 and 9 days of post spiking in Lake 1, Lake 2, Lake 3, Pond 1 and Pond 2 soil samples respectively (Figure 4). In second and third passage virus could not be isolated from the samples negative in the first passage. The viral RNA was detected by qRT–PCR up to 2, 20, 8, 9 and 9 days post spiking in Lake 1, Lake 2, Lake 3, Pond 1 and Pond 2 soil samples, respectively (Figure 4 and 5). Viral RNA could not be detected in Lake 1 soil on 3 and 4 DPS, in lake 2 soil on 21 and 22 DPS, in lake 3 soil on 9 and 10 DPS, in Pond 1 soil on 10 and 11 DPS and in Pond 2 soil on 10 and 11 DPS samples (Figure 5). The linear regression analysis indicated that the expected time required for virus to become undetectable was 3.33, 21.69, 9.96, 7.69 and 10.83 days against the observed values of 2, 19, 8, 6 and 10 days in Lake 1, Lake 2, Lake 3, Pond 1 and Pond 2 soil samples respectively. The coefficients of determination (R2) values for Lake 1, Lake 2, Lake 3, Pond 1 and Pond 2 soil samples were estimated to be 0.6, 0.868, 0.862, 0.858 and 0.928 respectively (Table 4 and Figure 6a–e).

Table 5: Coefficient of correlation (r) between soil parameters and viral persistence at 12°C and 25°C
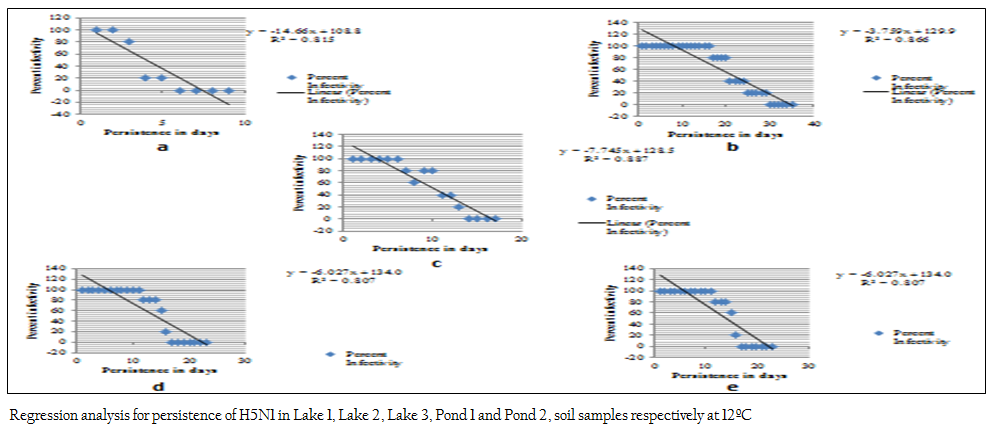
Figure 4: a–e: Regression analysis for persistence of H5N1 in Lake 1, Lake 2, Lake 3, Pond 1 and Pond 2, soil samples respectively at 12ºC
Coefficient of correlation (r) was calculated to study the effect of physico–chemical parameters of soil samples on the viral persistence at 12°C and 25ºC (Table 5). The strength of the correlation was estimated as per Cohen (1988). Among the soil parameters, soil pH showed a strong positive correlation at both the temperatures and whereas moisture content showed strong negative correlation at 12°C and moderate negative correlation on the viral persistence at 25°C. Critical values for the Pearson correlation was used to know whether the estimated coefficient of correlation (r) for each parameter is significant or not. None of the soil parameters were statistically significant at critical significance levels (α: alpha) of 0.05 but soil pH was statistically significant at critical significance levels (α: alpha) of 0.1
DISCUSSION
In the present study the mean persistence of HPAI virus in soil samples at 25°C and 12°C were 8 days and 15.4 days with ranges from 1–18 days and 5 to 29 days, respectively. Mean persistence of viral RNA in soil samples at 25°C and 12°C was 9.6 days and 16.6 days, respectively. HPAI virus (H5N1) persisted longer at 12°C as compare to 25°C indicating a strong negative correlation between the survivability of the virus and temperature. The survivability of the virus in the present study at 12ºC was, however, considerably lower than that observed by Nazir et al. (2011) who showed that T90 values (time required for 90 % loss of virus infectivity) for the avian influenza virus subtypes in sediment ranged from 43 to 54 days at 10°C.
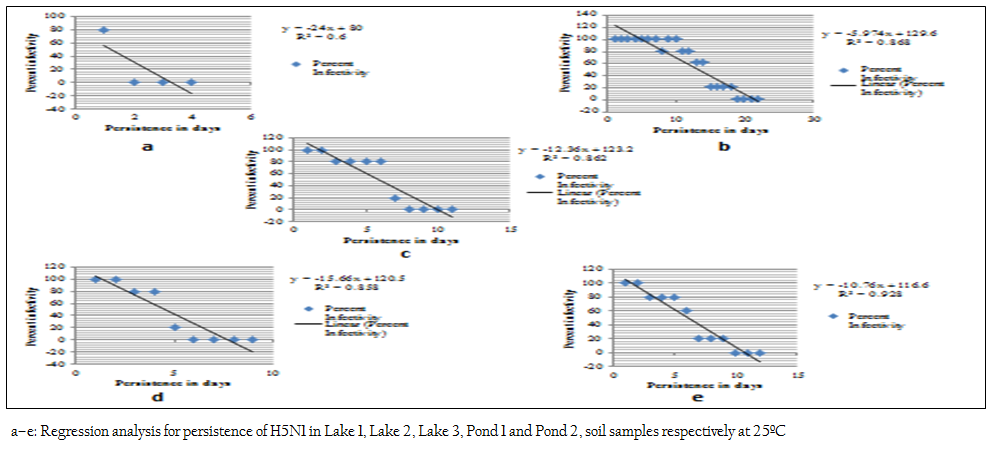
Figure 7: a–e: Regression analysis for persistence of H5N1 in Lake 1, Lake 2, Lake 3, Pond 1 and Pond 2, soil samples respectively at 25ºC
This variation may be due to variation between the virus subtypes and may also be due to compositional variation between the soil and lake sediment. The survivability of the virus in the present study at 25ºC was however, considerably higher than that observed by Horm et al. (2012) and Choi et al. (2009). Horm et al. (2012) studied the persistence of H5N1 virus in soil samples, they could not isolate the virus from the soil samples but they detected the viral RNA between 1–6 days from the lake mud at both 25°C and 32°C, between 1–14 days from the pond 1 mud at both 25°C and 32°C and between 1–14 days from the pond 2 mud at both 22°C and 32°C. Choi et al. (2009) used the vietnam isolate (A/Vietnam/1203/04) to study the persistence of H5N1 in soil coupons. They spiked the each test coupons with at least 1×107 TCID50 H5N1 virus. They were able to isolate the virus up to 24 hrs at room temperature (22ºC) with both low (40 %) and high relative humidity (80 %). In the present study pH showed a strong positive correlation on viral persistence at both 12°C and 25°C. The pH of the soil samples used in the present study ranged from 6.01 in Lake 1 to 7.83 in Lake 2. The persistence of AIV was least in Lake 1 soil sample(with pH 6.01) and was longest in Lake 2 soil sample (with pH 7.83) at both temperatures. This clearly indicates that acidic pH having negative effect on the viral persistence in soil samples at both temperatures. The effect of the pH on viral persistence in the present study was similar to those observed by Puri et al. (1990) and Sato et al. (1983). Puri et al. (1990) reported that members of the family Orthomyxoviridae are considered to be sensitive to acid pH values, although their retention of infectivity is dependent on degree of acidity and virus strain. Sato et al. (1983) reported that a low pH affects haemagglutinin protein which allows fusion with host cell membrane. The conformational change is reversible between pH 6.4 and 6 but irreversible below pH 5. Moisture content of the soil samples used in the present study ranged from 41.95 to 67.65 % and it showed a strong negative and moderate negative correlation on viral persistence at 12°C and 25°C, respectively. The effect of moisture content on viral persistence in the present study was contradictory to those observed by Hurst et al. (1980a) and Yeager and O'Brien (1979). Hurst et al. (1980b) reported that inactivation of poliovirus type 1, echovirus type 1, and indigenous entero viruses in the soil of a wastewater infiltration basin has been shown to be dependent on the rate of moisture loss, with drying cycles increasing virus inactivation. Yeager and O'Brien (1979) observed that drying of soil is virucidal to both poliovirus and Coxsackie virus and one of the mechanisms involved in poliovirus inactivation during drying seems to be the dissociation of the viral capsid and genome. In case of Lake 1 soil virus survived for short period even though it was having highest moisture content as compare to other soil samples and this may be due to lowest pH in the Lake 1 soil as compare to other soil samples. None of the soil parameters are statistically significant at level of significance (á) 0.05 and this may be due to low sample size. Higher numbers of observations are required to establish the significance of correlation between the physicochemical parameters and survivability at different temperatures. The study carried out gives an insight into the various physicochemical factors involved in the persistence of avian influenza virus in soil and this will help in better surveillance and epidemiological assessment of H5N1 virus in environment leading to better disinfection strategies in affected areas.
REFERENCES
Baleshwari K (2010). Persistence of Highly Pathogenic Avian Influenza virus (H5N1) in poultry faeces. M.V.Sc. thesis, submitted to Indian Veterinary Research Institute, Izatnagar.
Brown JD, Swayne DE, Cooper RJ, Burns RE, Stallknecht DE (2007) Persistence of H5 and H7 avian influenza viruses in water. Avian Dis. 51: 285 – 289.
http://dx.doi.org/10.1637/7636-042806R.1
PMid:17494568
Choi YW, Rogers JV, Chappie DJ, Taylor, Riggs KB, Willenberg ZJ (2009). Highly Pathogenic Avian Influenza H5N1 Virus Persistence Testing and Evaluation of Liquid Decontamination Technologies. Investigation and technology evaluation report U.S. Environ. Protect. Agency. 54: 1 – 23.
Cohen J (1988). Statistical Power Analysis in the Behavioral Sciences (2nd edition). Hillsdale (NJ): Lawrence Erlbaum Associates, Inc.
Fisher RA, Yates F (1974). Table VI. Statistical tables for biological, Agricultural & medical research, 6th edition London: Longman group ltd.
Hinshaw V, Webster R, Turner B (1979). Waterborne transmission of influenza A viruses. Intervirol. 11: 66 – 69.
http://dx.doi.org/10.1159/000149014
Horm SV, Deboosere N, Gutiérrez RA, Vialette M, Buchy P (2011). Direct detection of highly pathogenic avian influenza A/H5N1 virus from mud specimens. J. Virol. Methods. 176(1–2): 69 – 73.
http://dx.doi.org/10.1016/j.jviromet.2011.06.002
PMid:21683738
Horm VS, Gutierrez RA, Nicholls JM, Buchy P (2012). Highly Pathogenic Influenza A (H5N1) Virus Survival in Complex Artificial Aquatic Biotopes. PLoS ONE. 7(4): e34160.
http://dx.doi.org/10.1371/journal.pone.0034160
PMid:22514622 PMCid:PMC3325971
Hurst CJ, Gerba CP, Cech I (1980b). Effects of Environmental Variables and Soil Characteristics on Virus Survival in Soil. Appl. Environ. Microbiol. 40(6): 1067 – 1079.
PMid:6257161 PMCid:PMC291723
Hurst CJ, Gerba CP, Lance JC, Rice RC (1980a). Survival of enteroviruses in rapid–infiltration basins during the land application of wastewater. Appl. Environ. Microbiol. 40(2): 192 – 200.
PMid:6258471 PMCid:PMC291552
Liu JH, Okazaki K, Bai GR, Shi WM, Mweene A, Kida H (2004). Interregional transmission of the internal protein genes of H2 influenza virus in migratory ducks from North America to Eurasia. Virus Genes. 29: 81 – 86.
http://dx.doi.org/10.1023/B:VIRU.0000032791.26573.f1
PMid:15215686
McLean EO (1982). Soil pH and lime requirement. Agronomy. 9: 199 – 223.
Nazir J, Haumacher R, Ike AC, Marschang RE (2011). Persistence of avian influenza viruses in lake sediment, duck feces, and duck meat. Appl. Environ. Microbiol. 77: 4981 – 4985.
http://dx.doi.org/10.1128/AEM.00415-11
PMid:21622783 PMCid:PMC3147373
OIE Terrestrial Manual (2012). Avian Influenza. Chapter 2.3.4. p 6.
Puri A, Booy FP, Doms RW, White JM, Blumenthal R (1990). Conformational changes and fusion activity of influenza virus haemagglutinin of the H2 and H3 subtypes: effects of acid pre–treatment. J. Virol. 8: 3824 – 3832.
Sato SB, Kawasaki K, Ohnishi S (1983). Hemolytic activity of influenza virus hemagglutinin glycoproteins activated in mildly acidic environments. Proc. Natl. Acad. Sci. USA. 80: 3153 – 3157.
http://dx.doi.org/10.1073/pnas.80.11.3153
PMid:6574476 PMCid:PMC393998
Spackman E, Senne DA, Myers TJ, Bulaga LL, Garber LP, Perdue ML., Lohman K, Daum LT, Suarez DL (2002). Development of a real–time reverse transcriptase PCR assay for type A influenza virus. J. Clin. Microbiol. 40: 3256 – 3260.
http://dx.doi.org/10.1128/JCM.40.9.3256-3260.2002
PMid:12202562 PMCid:PMC130722
Stallknecht DE, Shane SM, Kearney MT, Zwank PJ (1990). Persistence of avian influenza viruses in water. Avian Dis. 34: 406 – 411.
http://dx.doi.org/10.2307/1591429
http://dx.doi.org/10.2307/1591428
PMid:2142420
Standards Association of Australia. AS 1289 B1.1–1977. Determination of the Moisture Content of a Soil: Oven Drying Method (standard method).
Webster RG, Yakhno M, Hinshaw VS, Bean WJ, Murti KG (1978). Intestinal influenza: replication and characterization of influenza viruses in ducks. Virol. 84:268 – 278.
http://dx.doi.org/10.1016/0042-6822(78)90247-7
World Health Organization (2002). WHO Manual on Animal Influenza Diagnosis and Surveillance. http://www.who.int/csr/resources/publications/influenza/whocdscsrncs.5.pdf.
Yeager JG, O'Brien RT (1979). Enterovirus inactivation in soil. Appl. Environ. Microbiol. 38: 694 – 701.
PMid:44178 PMCid:PMC243562